Symbiosis and its role in the evolution of eukaryotes
Back to main indexJump to section:
Introduction
We define symbiosis as the assembly of two or more organisms living in close association more or less continuously, independently of the relationships they have between them. Prey/predator relationships are therefore excluded. We can roughly define 3 main types of symbiotic relationships:
- Relationships where both partners have an advantage. We then speak of mutualism. In general, when we speak of symbioses without any other qualifier, it is this type of relationship that is implied
- Relationships where the two partners do not enjoy any obvious advantage, but neither are they troubled by any obvious disadvantage. We then speak of commensalism. We also speak of commensalism when one of the two partners does not derive any benefit from the association but the presence of the other does not bother him, as in the case of part of the microflora hosted by vertebrates. [Translator’s note: Very unclear how this is not mutualism]
- Relationships where one of the two partners takes advantage of the other, which suffers a loss of its biological capacities. We then speak of parasitism.
Endosymbioses are defined as an association where one of the two partners lives inside the other: the outer one is called the host and the inner one the endobiont. The most extreme case is when the symbiont lives inside the cells of its host. Besides these endosymbioses, there are ectosymbioses where the partners live in close physical association; most often the smallest symbiont or epibionte lives attached to its host. We have seen that there are also metabolic symbioses without any physical contact: syntrophy.
The association between the host and its symbiont can be facultative (non-obligatory), meaning that each of the partners can complete its life cycle independently of the other. On the other hand, the association may be obligatory for one, the other or both partners. In the case of parasites, of course, the host lives very well without its parasite. The converse is obviously often false: many parasites cannot live without their hosts! In the case of mutualism, all the combinations exist, with effects of the loss of the partner ranging from death to slight reduction in fitness. Even if they are small, these losses can still play a big role in the neo-Darwinian race for evolution.
Major types of symbioses can often be gradual, meaning that the same association can start as mutualism and end in parasitism and vice versa. Moreover, in the same phyletic group, there are often symbiotic species and other parasites. All mutualistic symbioses are in fact based on “balanced” interactions between the two partners, who derive mutual benefit from the association, or at least one of the two. However, these interactions are fragile and it often happens that one partner takes over the other. This is a first step towards a much less equitable relationship between partners. Conversely, eukaryotes have developed many defense mechanisms against parasites and in some cases parasite control results in a stable and mutualistic relationship, where the parasite provides, for example, new biosynthetic capacities. In fact, the data indicate that the same phenomena are involved, in particular at the level of recognition between the two partners in parasitic or mutualist interactions. Experimentally, it was possible in the laboratory to recreate the passage from a parasitic relationship to a more mutualistic relationship (Box 9). It only took a few months!
Box 9. Endosymbiosis in Amoeba proteus
There is an example of an endosymbiosis model in the laboratory which has made it possible to study the modalities of the establishment of a mutualistic endosymbiosis from a parasitic relationship. Indeed, K. Jeon was working on the amoeba species Amoeba proteus, a free amoeba of the Amoebozoa group. In his collection, he accidentally found a culture of amoeba infected with 'X' bacteria showing a marked decrease in their ability to proliferate. Analysis of the physiology and sequence of the rRNAs of these bacteria has shown that they are close to Legionella, obligate intracellular parasites. However, after 200 cell generations, or about 18 months, the amoeba resumed normal growth, even under certain conditions greater growth than those of uninfected amoebae. The bacteria of these amoebae are present in a special structure, 'the symbiosome'. The bacteria have been used to infect other amoebae either by injection into the cytoplasm or by endocytosis. While most are digested, a few survive and infect the amoebae. They then cause an acceleration of the growth of the amoebae for at least a year (the duration of the experiment)! In addition, X bacteria have become essential for the survival of the amoebae. Indeed, it is possible by microsurgery to remove the nucleus of amoebae and transplant it into a cytoplast, an cell devoid of a nucleus. Transplantation fails if the nuclei come from amoebae with symbionts and the transplanted cytoplast comes from an amoeba that lacks symbionts. It succeeds in all other experimental setups. The nucleus of these infected amoeba contains a lethal factor because when injected into an amoeba with a nucleus it dies! It is possible that this phenomenon is related to the presence of a protein of unknown function of about 30 kDa which is secreted in large quantities by the bacteria in the cytoplasm of its host. It is likely that this 'endosymbiosis' is not yet well stabilized, as the infected amoebae have become susceptible to heat, excess food and overpopulation. In the case of heat sensitivity, it has been shown that X bacteria are heat sensitive and that their death leads to that of the amoeba!
Mutualistic endosymbioses
We saw in the previous chapter that the mutualistic intracellular endosymbiosis at the origin of the mitochondria and the plastids were at the heart of the evolution of eukaryotes and are one of their major characteristics. In these two cases, integration is total and the symbionts have become integral parts of cells: organelles. Numerous mutualistic intracellular endosymbioses still occur today with a very high frequency and continue to allow additional adaptations. They are probably linked to the ability of eukaryotes to make endocytoses which facilitate access to the interior of the cell. In fact, only one example of bacterial/bacterial endosymbiosis is known with the presence of a eubacterium inside another eubacterium (see pages 20 & 21). The inventory of endosymbioses involving eukaryotes is far from complete (see Box 10 for examples of prokaryotic/eukaryotic symbioses and Box 11 for eukaryotic/eukaryotic symbioses). We do know, however, that virtually all groups of eukaryotes have the ability to carry symbionts. The reason is that for the endobiont, the intracellular medium is rich in nutrients, hence also the coexistence of mutualistic organisms and parasites in the same groups. Another reason is protection from infections. For the host, the advantages are of various kinds: ability to synthesize carbonaceous materials, amino acids, toxic secondary metabolites or to fix nitrogen…
Box 10. Endosymbiotic prokaryotes
Rhopalodia gibba and Epithemia turgida are diatoms (Bacillariophyta) that harbor 'spherical bodies' in their cytoplasm, which are derived from cyanobacteria. Similar spherical bodies have also been discovered in Braarudosphaera bigelowiides, distant coccolith algae of the Haptophyta group. The cyanobacteria are closely related to free cyanobacteria of the genus Cyanothece, but cannot be grown outside the host cell. Spherical bodies have three membranes and are transmitted faithfully during divisions. The genome of the Epithemia turgida endobiont no longer codes for a photosynthetic system, has lost some biosynthetic pathways and contains many pseudogenes. They are therefore more organelles than endobionts. In fact, as eukaryotes are not able to fix nitrogen, there are many symbioses with nitrogen fixing cyanobacteria in other protists, fungi, invertebrates... The most famous is between the bacteria of the genera Rhizobium and legumes or Frankia and other eight families of plants, including Rosaceae.
Rhizopus microsporus is a Mucoromycotina fungus that destroys young rice shoots and can cause severe yield losses. In fact, this fungus lives in symbiosis with a bacterium of the genus Burkholderia which produces a polyketide toxin, rhizoxin. This toxin is responsible for destroying the rice shoots. The bacteria are transmitted by spores but can be cultivated independently of the fungus. When the fungus is rid of its bacteria, it becomes unable to form sporangia. The reintroduction of bacteria makes the fungus again able to differentiate these structures. To get inside fungal hyphae, bacteria do not use endocytosis. The genome sequence of the bacteria shows that it has started to adapt to its host because it codes for many transporters that allow exchanges with its host; it would import citrate which would serve as a carbon source and would export amino acids and co-factors. Several other fungi live in symbiosis with intracellular bacteria, including Glomeromycota, Mucoromycotina and Pezizomycotina. We so far do not known the advantages of these symbioses.
There are many other examples of bacteria conferring the ability to produce toxins. For example, many Dinoflagellata carry endobiont bacteria which appear to be the source of the toxins they excrete. Finally, in the paramecia (Ciliophora), bacteria transmitted with the cytoplasm during sexual reproduction produce toxins, the kappa particles, that kill the paramecia devoid of bacteria.
The cyanobacterial symbionts of the Glomeromycota Geosiphon pyriforme are an example of nutritional endosymbiosis of the 'plastid' type, independent of the main primary endosymbiosis and visibly less successful. In the case of Geosiphon pyriforme, cyanobacteria of the genus Nostoc can live independently of the fungus, while the latter requires cyanobacteria to survive. Bacteria are not transmitted by the spores during the reproduction of the fungus, which must re-associate after germination of the spores. The cyanobacteria fix not only CO2 but also N2, which allows a complete autonomy of this consortium.
Bacterial symbioses are beginning to be very studied because they could help in the fight against certain diseases, in particular in insects and nematodes, or help improve the yields of carbon dioxide fixation in the case of associations with mycorrhizal fungi or plants. Indeed, in some cases, the association is mandatory and the death of bacteria leads to the disappearance of the host. This seems to be the case, for example, in filaria: nematodes responsible for filariasis such as elephantiasis. Treatment with antibiotics is then enough to get rid of the parasite!
Analysis of current intracellular endosymbioses shows more or less successful integrations between the two partners. However in many cases one or both partners cannot live alone. The question whether to consider the symbiont/host pair as one or two separate organisms then arises. So much so that this leads several researchers to consider that intracellular endosymbiosis are a much more important engine of evolution than genetic mutations because they lead to new organisms with radically different properties. These same researchers therefore question, and probably rightly so, the unique use of molecular phylogenies to establish kinship relationships between eukaryotes. However, the final establishment of the symbioses goes through classical neo-Darwinian-type processes of mutation and selection, allowing efficient transmission of the endobiont (seeFigure 45for an example where this problem has not been completely resolved) and adaptations allowing the full expression of selective advantages. For example, in the case of symbiotic algae which must ensure photosynthesis, the alga must export nutrients and the host must not have tissues that are too opaque for light to penetrate, while protecting themselves from UV rays. This limits, for example, the development of protective shells. Some hosts produce substances that effectively absorb UV, in other cases, it is synthesized by algae. The host must also channel the oxygen produced by photosynthesis to prevent it from causing too much damage to its tissues. These processes probably require several mutations in the host and in the endobiont. Recent data show that in the case of bacteria, the mutations involved could occur very quickly. Indeed, a culture of Salmonella in a rich medium made to resemble intracellular content, and with population bottlenecks to mimic selection events, shows that these bacteria lose DNA at the rate of 0.05 bp/generation. The modification of the genome is therefore complete in a few weeks. This most often occurs as deletions up to 200 kb in size. In mutS mutants affected in repairing DNA mismatches, this rate is 50 times higher. The establishment of the symbiont’s dependence on its host and vice versa can therefore occur in very short periods of time with regard to evolution.
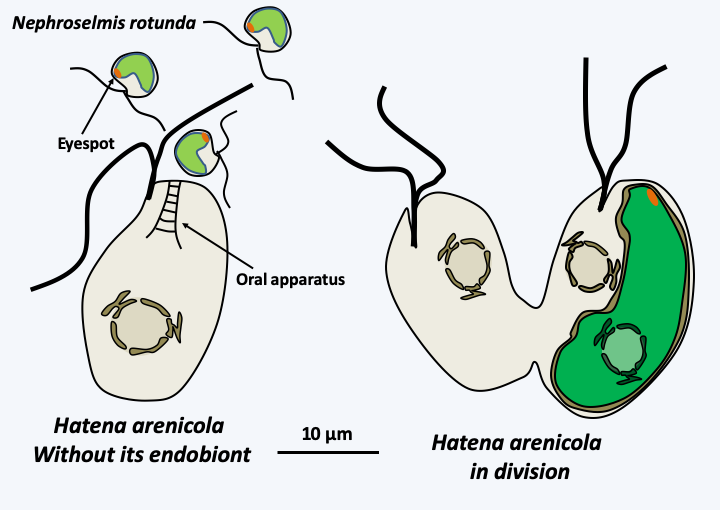
Figure 45.
The segregation of the photosynthetic endobiont of Hatena arenicola. The katablepharid Hatena arenicola, has not completely resolved the segregation of its endobiont, an intracellular Viridiplantae that has retained its nucleus and inner membrane network and resides in a symbiosome with a single membrane. This alga belonging to the genus Nephroselmis has a plastid ten times larger than that of individuals living freely and does not divide. The flagellate, when it divides (right), therefore gives rise to one cell with the endobiont and the other without. The algae-free form then differentiates an 'oral' apparatus which allows it to phagocytose free algae (left). If the algae is of the correct species, its plastid enlarges and the 'oral' apparatus regresses. After transformation, the flagellate becomes dependent on its algae for its food, but still seems capable of phagocytizing prey.Box 11. Endosymbiotic eukaryotes
Many organisms have endobiont algae called zooxanthellae or zoochlorella in their cytoplasm. Zooxanthellae are Dinoflagellata of the genus Symbiodinium: endobionts of invertebrates and marine protists. These algae are widely studied in the context of coral biology, because their presence is necessary for the survival of these cnidarians. Zoochlorellae are Viridiplantae of various genera (Choricystis, Elliptochloris, Auxenochlorella, Chlorella, Scenedesmus, etc.): endobionts of freshwater and marine invertebrates or protozoa. It is often possible to cultivate both mates independently, but in nature the association often seems obligatory. Transmission of zooxanthellae and zoochlorella is not always effective. Indeed, many invertebrate larvae do not receive symbionts from their parents and acquire them a posteriori by endocytosis. The algae often have a broad host spectrum and, conversely, a single host can have several types of endobionts. Paramecium bursaria, a Ciliophora, for example harbors algae of five different species.
The main benefit conferred to the host is the supply of carbonaceous nutrients. It is known that in some chlorellae, up to 40% of the products of photosynthesis are exported. In return, the host provides a certain amount of waste that serves as fertilizer for the algae. This syntrophy allows the couple to grow in poor environments. Several researchers have suggested that the bleaching and death of corals may be due to an effect of increased temperature and/or UV light on zooxanthellae.
Fungi often live in symbiosis. However, in general, these symbioses are not endocellular and the rare intracellular fungi are usually parasites. Nevertheless, a particular endosymbiotic relationship between a yeast dividing by fission and a sponge (Chondrilla sp.) has been demonstrated. Sponges contain many prokaryotic and eukaryotic symbionts, most often between their cells, which is the case with this yeast, living in the interstitial tissue of the sponge but also inside cells, apparently directly in contact with the cytosol. At the time of reproduction, the yeasts are transmitted vertically via the oocytes. Little is known about this symbiosis other than the fact that, when pulled from their environment, the yeasts die off quickly, suggesting an obligatory symbiosis.
Other mutualistic symbioses
The other mutualistic symbioses are as frequent and important as the endosymbioses and have probably made possible very important events for the biosphere. The conquest of the terrestrial environment by photosynthetic organisms has occurred at least twice thanks to these symbioses: the first time by algae in association with Eumycota fungi to give rise to lichens (Figure 46) and a second time by multicellular Viridiplantae in association with Eumycota, in particular, Mucoromycotina and Glomeromycota, to give the rise to land plants (Figure 47).
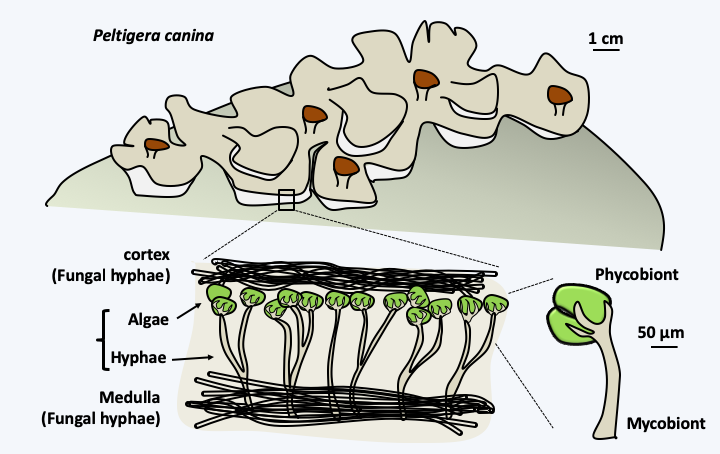
Figure 46.
Structure of lichens. Lichens, such as Peltigera horizontalis, are mutualistic symbiotic organisms formed by the association of a fungus or mycobiont with algae, cyanobacteria such as in Peltigera canina, or both: phycobionts. They are perfectly adapted to terrestrial life and have colonized many surfaces. The hyphae of the fungus surround the algae, sometimes very intimately via haustoria, but do not penetrate algal cells. As with plastid-type endosymbiosis, the phycobiont provides carbonaceous matter, or even fixes atmospheric nitrogen if the symbiotic cyanobacteria has the capacity. What the fungus provides is less clear, possibly protection, mineral salts and the conquest of terrestrial biotopes. The combination is often mandatory for the fungus, while it is optional for algae. Nevertheless, free algae are generally rare in nature.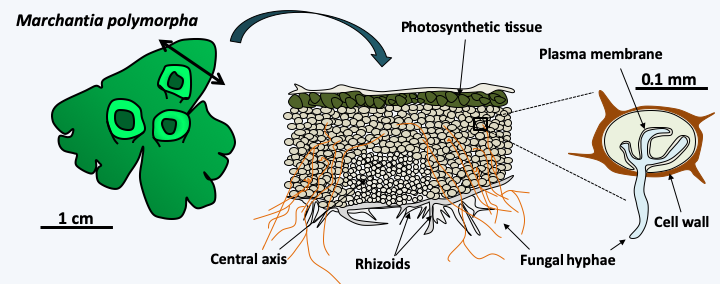
Figure 47.
Presence of endomycorrhizae in Marchantia polymorpha. Mycorrhizae are mutualistic associations between plants and fungi (see Box 13). The liverwort Marchantia polymorpha is one of the liverworts that diverged from other plants the earliest in evolution. Like almost all other plants, it involves endomycorrhizae with fungi, from the Glomeromycota group for this species. On the contrary, no current algae is a partner in such symbiosis, suggesting that the association with fungi was one of the key events that allowed the release of water by the ancestors of plants. The fungus penetrates the wall of the plant cell, but not the plasma membrane.Some researchers believe that the flagellum, whose complexity is so important, could only appear thanks to ectosymbiosis with a bacterium, like what exists in certain flagellates present in the intestines of termites that have epibiont bacteria allowing movement (Figure 48). In other protists, epibiont bacteria participate in defense reactions (Figure 49).
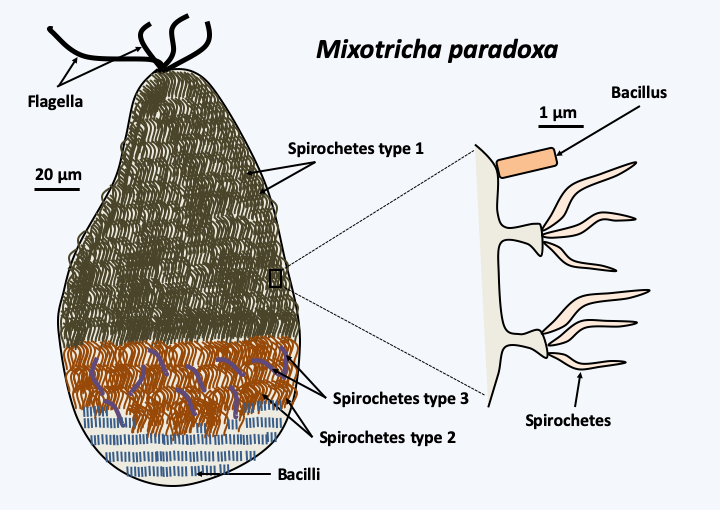
Figure 48.
Mixotricha paradoxa and its epibionts. The flagellate Mixotricha paradoxa lives in the digestive tract of termites and has long surprised biologists. Indeed, its first description in the 1930s indicated that it used to move four flagella located at the anterior pole as well as numerous cilia. More recent studies have shown that the cilia are in fact epibiontic spirochete bacteria. Electron microscopy analyses and molecular identification of bacterial species have shown that at least four different types of bacteria live attached to Mixotricha paradoxa. Bacilli uniformly cover the flagellate. They are clearly visible at the posterior pole devoid of the other epibionts. Three types of spirochetes are present in well-defined regions. Type 1 at the anterior pole and types 2 and 3 in a band surrounding the middle of the cell. Bacteria ensure the mobility of Mixotricha paradoxa; the flagella serve only as a rudder. They prevent too rapid evacuation of the protozoan by the flow of liquid in the digestive tract.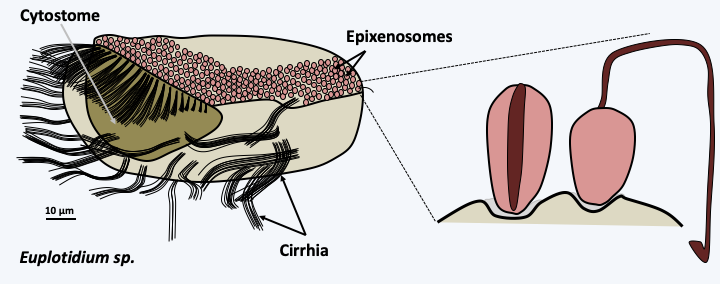
Figure 49.
Euplotidium and its epixenosomes. Ciliates of the genus Euplotidium are predators feeding on microscopic algae that they phagocytose via their cytostome or oral apparatus. They move with the help of cirrhia formed from aggregated cilia. They themselves are the prey of another ciliate, Litonotus lamella. To defend themselves against this predator, the Euplotidium use epixenosomes present on their dorsal surface. Upon contact with the predator, the epixenosomes extrude a long tube terminated by a hook (right), preventing phagocytosis. Epixenosomes are bacteria from the Verrucomicrobia group. Only Euplotidium without epixenosomes are efficiently phagocytosed by Litonotus lamella. Other protozoa and various algae have repeatedly evolved oragnelles from cellular structures, with the same function as epixenosomes: trichocysts or ejectosomes.Parasitism
As important in number as they are as a motor of evolution, parasitic relationships are ubiquitous in the biosphere. Virtually every eukaryote has several prokaryotic, eukaryotic (Figure 50) and viral parasites; the parasites themselves not being free from parasites, the hyperparasites (Figure 51)! Parasitism appeared by convergent evolution in practically all groups of living beings. There are two types of parasites: virulent and opportunistic. Virulent parasites have a strong host invasion capacity and high infectivity which is measured using the 50% lethal dose, i.e. the dose of parasites injected at which half of the hosts die. They also show significant pathogenic potential, i.e. an ability to destroy host tissue, escape defense systems or produce toxins. Often these parasites have a low host spectrum that they can successfully infect. The relationships they maintain with their host more or less quickly become obligatory and they are never found in the environment other than in the form of dispersal propagules (spores or cysts). The ultimate stage is when they can no longer grow apart from their hosts and have therefore become obligate parasites. They have then very often lost a large part of their initial virulence. Opportunistic pathogens can only develop if the host is weakened, which is the case for example in immunocompromised individuals, but also for example trees injured in storms. These organisms do not have clear strategies for attacking hosts, but take advantage of either weak defense responses by the host, or entry points into the healthy host such as injury. In contrary to virulent parasites, opportunistic ones often have a broad host spectrum and are frequently found in the environment in free form.
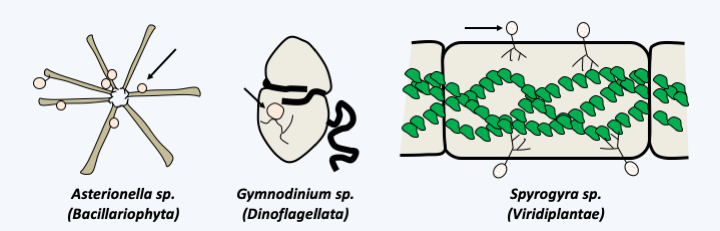
Figure 50.
Three algae parasitized by fungi. Parasitic fungi are chytridiomycetes (arrows), which are aquatic fungi that disperse using flagellated spores (zoospores). These fungi are frequent pathogens of algae but also of various other protists, even cyanobacteria.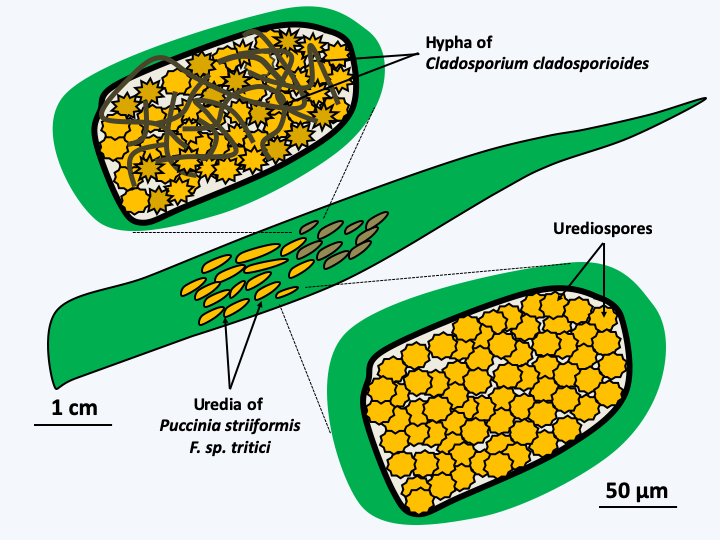
Figure 51.
Hyperparasites of wheat yellow rust Puccinia striiformis f. sp. tritici. On a wheat leaf, the yellow rust produced uredias containing spores. Yellow rust is an obligate pest that cannot be cultivated without its host. The uredias on the right are parasitized by a hyperparasitic yellow rust fungus Cladosporium cladosporioides. This fungus also lives as a saprotroph and is frequently found indoors. It is therefore an opportunistic pathogen of yellow rust. There are at least three others: Lecanicillium lecanii, Microdochium nivale and Typhula idahoensis. These hyperparasites are being considered as alternative treatments to antifungals to get rid of yellow rust, which can cause serious losses in crop yield and quality.Parasites have developed often extremely complex strategies to complete their life cycle. If they reach sexual maturity in the so-called final host, it is common for them to go through one or more intermediate hosts, where each time they must have repeatedly implemented complex and effective developmental programs. Indeed, in order to be able to successfully invade a host, the parasites must recognize the right host and attach themselves firmly to it. For endoparasites and especially intracellular parasites, it is necessary to penetrate the host organism and survive the defenses it has put in place. Finally, nutrients must be pumped efficiently in order to reproduce and have the means to transmit enough offspring to other hosts. Obviously, the hosts implement countermeasures at each of these stages, such as mechanisms to avoid places or individuals carrying the parasites. The person who understood how “germs” work has, for example, developed hygiene and quarantine. Note that these methods are general in the living world. For example, ants quickly evacuate the corpses of ants killed by fungi that infect them. Other strategies include strengthening protection by thickening the cell walls, secretion of secondary metabolites - e.g. plant tannins - reducing the proliferation of parasites, improvements in systems for detecting and destroying invaders such as innate and non-innate immune systems found in animals, the hypersensitive and systemic responses of plants, the mechanisms of antibiotics and hyphal interference in fungi, or the trapping of nutrient reserves in forms not available for parasites. This is the case, for example, with iron which is often very strongly bound to storage proteins. For most microorganisms, the internal environment of mammals is deficient in iron.
Host/parasite evolution has come to be explained by the “Red Queen hypothesis”, in reference to Alice in Wonderland by Lewis Carroll, where Alice and the Queen of Hearts are on the spot: for each measure, a counter measure is needed so as not to disappear from the race sanctioned by natural selection and which will be won if the number of descendants produced is sufficient to sustain the species or the population. In other words, you need to constantly move, or evolve, to stay in the same spot. In the case of host/parasite relationships, this is particularly crucial and requires constant and rapid changes because the slightest misstep quickly results in the elimination of one of the two partners. These processes are very well-studied in cases of parasitism because of the medical and agricultural repercussions, much more than for the mutualistic relations, which however bring into play the same molecular mechanisms. Note that if we have a wide range of antibiotics and vaccines to fight effectively against parasitic bacteria, the same is not true for eukaryotic parasites, where the pharmacopoeia is often reduced and vaccines often ineffective. In Western societies, the main parasites are bacterial or viral in nature, however this is not the case in the tropics, where parasitic protozoa play a very large role, or in other organisms (in plants and insects, the major parasites are fungi).
Back to chapter index