Alveolata
Back to main indexJump to section:
Introduction
The Alveolata have been clearly defined thanks to molecular phylogenies. The phylogenies indicate that Alveolata are related to Stramenopila and Rhizaria in a lineage called SAR or Harosa (Figure 215). Alveolata have the common characteristics of having vesicles positioned just under their plasma membrane, forming a kind of alveoli (Figure 294). Their mitochondria have tubular ridges. The alveoli have allowed the evolution of attributes specific to each evolutionary lineage. Note that the Glaucophyta also have alveoli which probably appeared by evolutionary convergence.
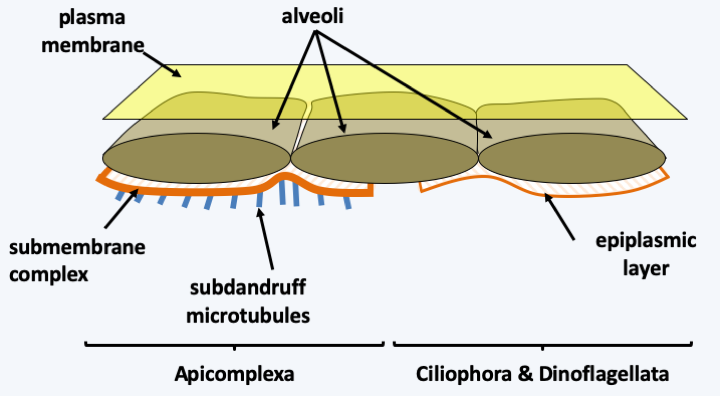
Figure 294.
Sub-membrane alveoli in Alveolata. The alveoli are associated with a submembrane protein complex and a network of microtubules in Apicomplexa. They are associated with a fibrous layer called the epiplasmic layer in Ciliophora and Dinoflagellata.Molecular phylogenies distinguish 3 main groups of Alveolata: Ciliophora, Apicomplexa and Dinoflagellata, each having followed a very particular evolutionary process (Figure 295). In addition to these three groups, there are a few minority lines. Note that analyzes of marine picoplankton show that two other as yet unstudied groups of Alveolata exist. These two groups appear to be the major components of picoplankton. Their biology is still completely unknown to us.
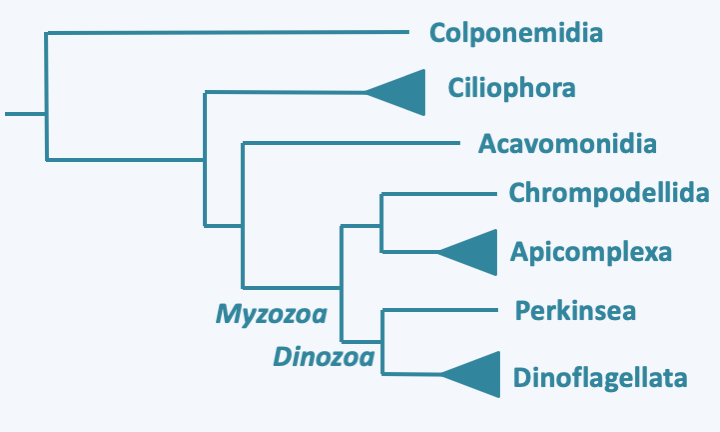
Figure 295.
Phylogeny of Alveolata.Plastids have clearly been present during evolution of the Apicomplexa and Dinoflagellata, but if they were present also in Ciliophora is currently under discussion. Plastids are functional in most Dinoflagellata and have undergone a complex evolution with multiple replacements. They regressed in Apicomplexa, to the point of disappearing completely in some species. Independent regressions were also identified in other Alveolata, which leaves the reason for their absence in Ciliophora unanswered. According to some biologists, these organisms have always been devoid of the plastid and it is the result of a secondary endosymbiosis or kleptoplasty that occurred in the ancestor of the Apicomplexa/Dinoflagellata (Myzozoa; see Figure 40). The predatory members of this lineage are currently capable of myzocytosis, which supports this hypothesis. In this context, the plastid, although also derived from a Rhodophyta, would have a different origin from that of Stramenopila / Haptophyta / Cryptophyta. Another group of biologists supports a common origin to the plastids of Alveolata and those of Stramenopila / Haptophyta / Cryptophyta. This hypothesis brings more complex scenarios of evolution into play. For instance, the plastid would have to have been lost among the Ciliophora. The plastid would have have been kept in the lineage leading to the Apicomplexa, in which it has four membranes, and in the Dinoflagellata, it would have been lost and replaced several times.
Ciliophora
The first diverging Alveolata group is the Ciliophora phylum, commonly known as the ciliates. Their origin is ancient because fossil ciliophora thecae date back to 450 Ma ago. The phylum includes about 7,000 species of single-celled phagotrophs which occupy many ecological niches. There are planktonic and benthic forms, in the sea or in freshwater; others live in moist soils, mosses, etc. A few species live with various symbionts: algae and bacteria. The algae provide carbonaceous matter and the bacteria toxin “killers” which kill individuals who do not harbor them. Some species of Ciliophora are parasites, others are commensal and live in the intestines of various herbivorous mammals. One species, Balantidium coli, occasionally parasitizes humans (Figure 296). A few species, such as Ichthyophthirius multifiliis, are fish parasites and can wreak significant havoc in fish farms or aquariums. In nature, Ciliophora are often the second link in the food chain in ecosystems. They feed on bacteria, algae or other protozoa that they ingest through their cell mouth or cytostome. Experiments have clearly shown that their presence keeps the amount of bacteria in many biotopes low, as they consume up to 90% of bacteria and algae. In the laboratory, it is possible to cultivate the species of the genus Tetrahymena on simple media with nutrients in solution, while members of Paramecium demand living bacteria and Stentor feed on other Ciliophora. Note that the macronuclear genome sequences of Paramecium tetraurelia and Tetrahymena thermophila did not identify genes typically functioning in plastids, suggesting that if the ancestor to these organisms did have a plastid, it did not leave genomic traces.
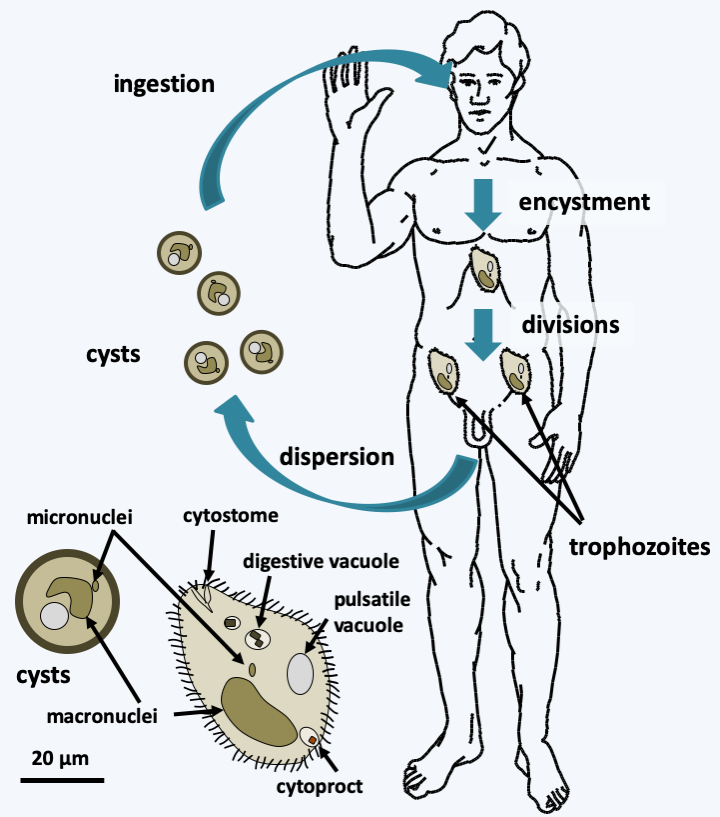
Figure 296.
Structure and cycle of Balantidium coli. This species has a simple life cycle. The ciliated trophic form, having the typical cellular structure of Ciliophora, lives in the intestine and feeds on the intestinal contents and cells of the intestine, in which it produces lesions. Attack on the intestinal mucosa causes dysentery that is rarely fatal. Resistant and infectious cysts allow survival in the environment and are spread through contaminated food. Its reservoir is the pig.Most Ciliophora are large in size; Stentor measures up to 2 mm. Ciliophora are very complex with defined morphologies that vary depending on the species. Each cell resembles a “little animal” with a musculoskeletal system, environmental sensing systems and a digestive system comprising a mouth, an intracellular stomach and an apparatus for excreting waste or cytoprect (Figure 296andFigure 297). They are polarized with the presence of a front and a rear. However, the two main characteristics of Ciliophora are the presence of a complex and important ciliature, which gave them their name, and especially the presence of two different types of nuclei, called micronuclei and macronuclei. The ciliature allows rapid movements and facilitates the capture of prey. Micronuclei are diploid and serve only for the transmission of information during reproduction. They are transcriptionally inactive and can be considered “germline” nuclei. Micronuclei have chromosomes and resemble nuclei of eukaryotes in general. They are divided by classical mitoses. Macronuclei, on the other hand, carry fragmented chromosomes and are transcriptionally active. If they divide, it is through a process of partitioning DNA molecules that is not mitosis. The micronuclei therefore form a lineage of germ nuclei specifically devoted to reproduction, while the macronuclei mimic a somatic line and ensure the expression of genetic material. Macronuclei disappear either during cell division or during sexual reproduction. They are resynthesized from micronuclei by modifications of genetic material, in ways that call for epigenetic information (Box 21). Note that depending on the species, there are one or more micronuclei and one or more macronuclei per cell. This particular biology of the nuclei has been accompanied by modifications of the nuclear genetic code in greater proportions than in other organisms. For example, UAA and UAG code for glutamine instead of stop in Tetrahymena, Paramecium and Stylonychia. UAA codes for glutamic acid in Vorticella. The most surprising is found in Euplotes where UGA codes for cysteine or for selenocysteine. Which amino acid is inserted depends on signals present in the messenger RNA, the two amino acids can be inserted via separate UGA codons in the same mRNA! The Ciliophora divide by transverse fission (Figure 297). Their polarization therefore involves complex morphogenetic mechanisms, as the front part must regenerate a rear and vice versa. Some of the morphogenetic processes are inherited in a non-Mendelian way (Box 21). Some species are able to differentiate into a cyst when conditions are unfavorable and to “sleep” for several months. The sexual cycle has been described in many species and follows modalities that are specific to Ciliophora (Figure 297). Often there are systems of mating types that determine compatibilities between cells. These are also sometimes inherited epigenetically (Box 21). Sexual reproduction is necessary for the survival of many Ciliophora clones. Indeed, the life of a clone is divided into three phases which can last up to several hundred divisions. Right after conjugation, there is a first period of immaturity during which the cells divide vigorously but cannot conjugate. After that, a phase of maturity is established where the cells divide vigorously and can conjugate. Finally, there is a phase of senescence where growth slows down and the cells end up dying unless they conjugate or self-fertilize. The first phase is controlled by the production of a protein called “immaturin”. The transition from immaturity to maturity is controlled by processes of gene repression / activation, the details of which are still unknown. The senescence phase appears to be controlled by changes in the macronucleus because the injection of macronuclei from young cells into older cells significantly prolongs the life of the latter.
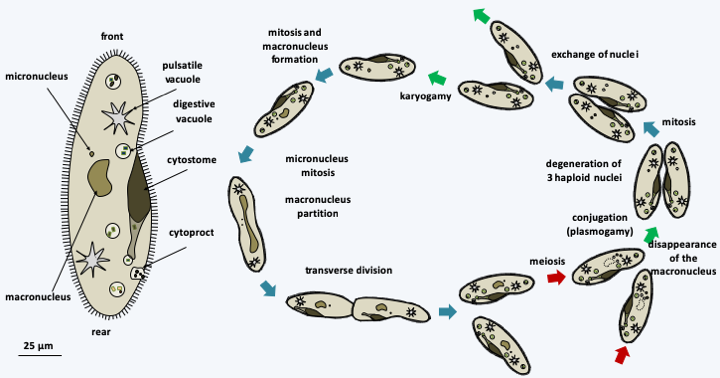
Figure 297.
Structure and cycle of Paramecium caudatum. In this species, there is only one micronucleus and one macronucleus; the cell shows the typical cell structure of Ciliophora. It divides by transverse fission after mitosis of the micronucleus and the partition of the macronucleus in two . During sexual reproduction, two compatible cells join together and create a cytoplasmic bridge. Their macronuclei disappear. The micronuclei undergo meiosis and three of the four haploid nuclei degenerate. The remaining nucleus undergoes mitosis and one copy remains in its cytoplasm and the second migrates into the cytoplasm of the partner cell. The haploid nuclei fuse; the process therefore results in two cells which have exactly the same genetic information in the diploid zygotic nucleus but which have different cytoplasm. At the end of sexual reproduction, after mitosis of the zygotic nucleus, a new macronucleus is reformed from one of the two diploid micronuclei. In some homothallic species, the process occurs with a single partner and the two nuclei resulting from postmeiotic mitosis merge; this process is called autogamy.Long based on morphology, ciliary structure and other ultrastructural features, the classification of Ciliophora has been improved by DNA sequence data. Molecular phylogenies currently define two sub-branches (Figure 298).
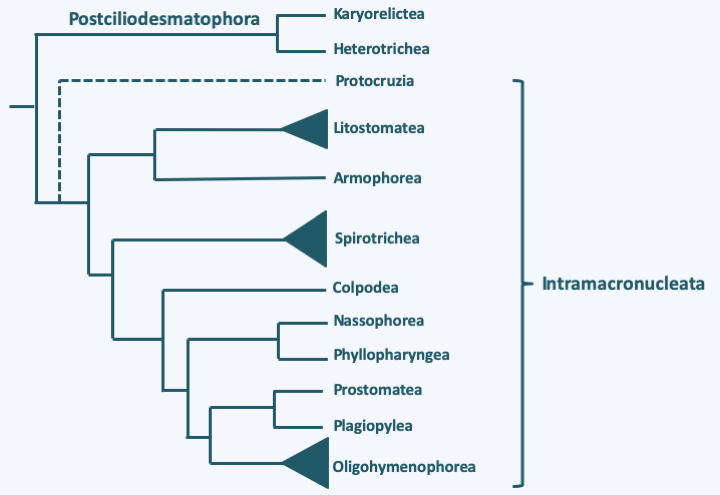
Figure 298.
Phylogeny of Ciliophora.Postciliodesmatophora
The Postciliodesmatophora subphylum contains two classes, Karyorelictea and Heterotrichea, whose members exhibit characteristics that appear to be more “primitive” than those of other Ciliophora. Members of the two classes exhibit common structures in the basal body environment, in particular the presence of a ribbon of specific microtubules attached to the basal body called “postciliodesmatum”, confirming the results of molecular phylogenies.
Karyorelictea
There are about 130 species of Karyorelictea that live mainly in coastal sediments; only the genus Loxodes contains freshwater species (Figure 299). The most notable feature of members of this class is the non-dividing macronucleus which has reduced ploidy; in some cases it even seems to remain diploid. Macronuclei are segregated at random during divisions and eventually degenerate. They must therefore be constantly produced from micronuclei. These groups of Ciliophora cannot be cultivated in the laboratory. Some can grow very large, sometimes close to half a centimeter. They have colonized many marine and freshwater environments.
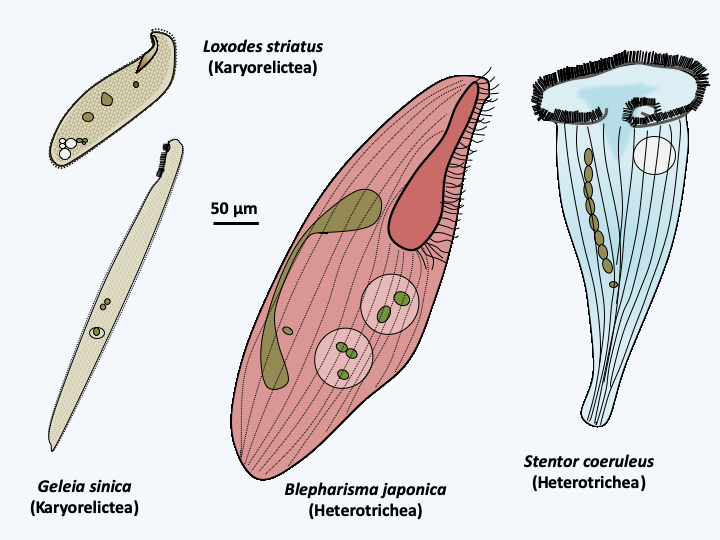
Figure 299.
Some Postciliodesmatophora.Heterotrichea
There are several hundred species of Heterotrichea (Figure 299). Like Karyorelictea, species of Heterotrichea are usually large, and characterized by division of the macronucleus with the help of microtubules located outside the nucleus. Their name derives from the fact that they have long cilia involved in the formation of a “paraoral” membrane around the cytostome, and smaller cilia on the rest of the cell body. Their body is very deformable; Stentor can, for example, retract into a spherical shape. Some have beautiful red or blue colors.
Intramacronucleata
The second subphylum, that of Intramacronucleata, includes the vast majority of Ciliophora. All members are characterized by the presence of microtubules inside the macronucleus during its division. The subphylum is made up of three major classes and seven minor lineages. These lines are differentiated mainly on the structure of the cilia and are generally confirmed by the sequence data. Nevertheless, convergences in the arrangement of the cilia or the structure of the basal bodies have been identified, making molecular phylogenies important for obtaining a better phylogenetic classification. A first line, containing only one genus, Protocruzia, and a few species, seems to diverge first. However, molecular phylogenies provide conflicting data as to its exact position in the Ciliophora tree and this genus could possibly belong to the Postciliodesmatophora subphylum. These are marine and benthic organisms (Figure 300) whose macronuclear behavior is different from that of other Intramacronucleata, confirming that it could be an early-diverging group, as shown inFigure 298. Indeed, they have a single micronucleus surrounded by ten or so macronuclei. These divide by a process that resembles true mitosis with condensation of the chromosomes and an anaphase-like separation.
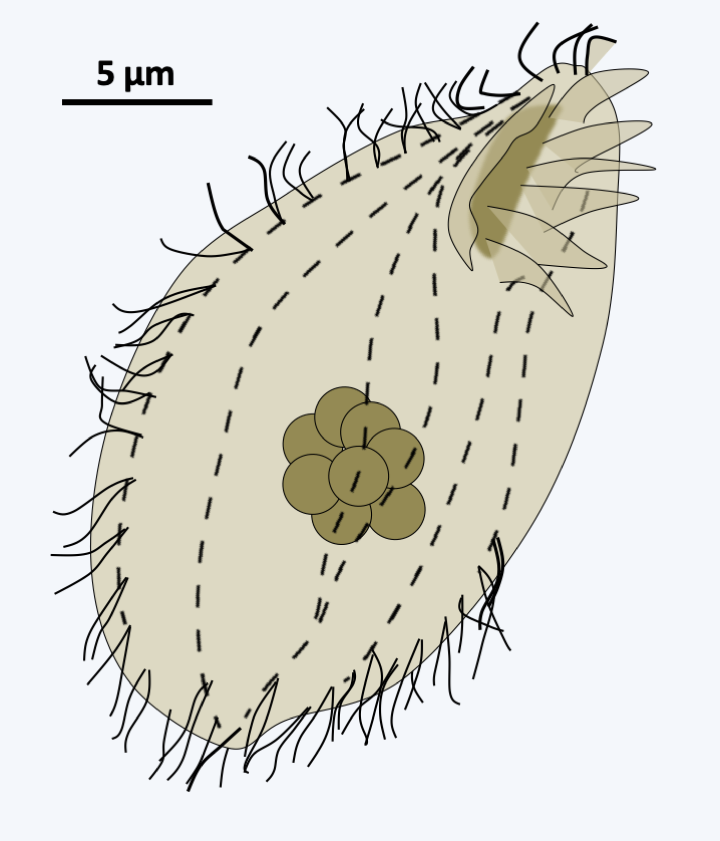
Figure 300.
Protocruzia contrax.Listostomatae
The major class of Intramacronucleata that appears to diverge first is Listostomatae (Figure 298). It contains several hundred species, some of which live free and others in commensal, parasitic or mutualistic symbionts of the vertebrate intestines. The human parasite Balantidium coli (Figure 296) belongs to this class. Some species appear to have a particular diet involving kleptoplasty (see Box 7). Others are very voracious and consume flagellates, ciliates, and even small animals. The sizes vary from about 30 microns to almost 2 mm and the shapes of these Ciliophora are very diverse (Figure 301).
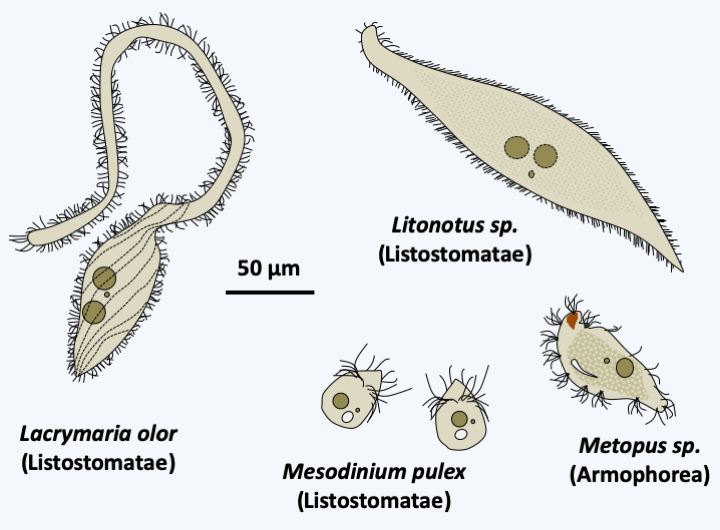
Figure 301.
Diversity of Listostomae and Armophorea.Armophorea
The class of Armophorea is related to that of Listostomatae (Figure 298). It brings together a few hundred species of anaerobic Ciliophora, whose mitochondria have evolved into hydrogenosomes. They thrive in marine or freshwater sediments, the water column of anoxic lakes or in symbionts in the intestines of various animals including ruminants. Some of them harbor methanogenic bacteria. They feed on bacteria and excrete acetate and propionate, which in symbiotic species can be consumed by the host. Most species have the ability to differentiate cysts for their survival or transmission. They have an average size for Ciliophora, i.e. around 100 μm (Figure 301). The shapes adopted vary widely: ovoid, elongated, helmet-shaped, etc.
Spirotrichea
The second important class which then diverges is that of Spirotrichea (Figure 298). With a few thousand species, this class is very diverse with few characteristics that bring the different members together (Figure 302). In particular, the morphology is very variable and the sizes vary from 5 μm to 1 mm, even if most of the members are between 100 and 200 μm. Part of the species are protected in a lorica. The macronuclear genome is often extremely fragmented, a feature they share with Phyllopharyngea and Armophorea, but which appears to have been acquired through convergent evolution in all three classes. The area around the cytostome usually shows structures resembling the paraoral membrane of Heterotrichea, a feature also acquired by convergent evolution. The ciliature is often sparse and the cilia can be grouped together in cirrhia. Some of the better known ciliates such as Euplotes and Stylonychia belong to this class (Figure 302).
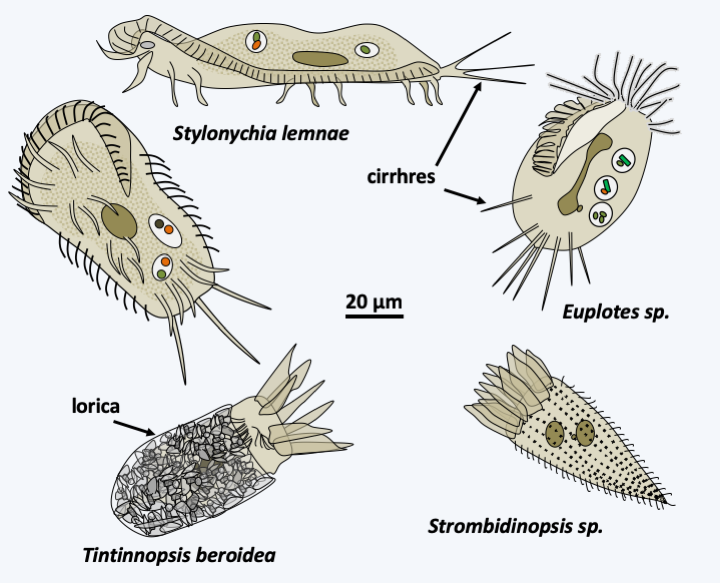
Figure 302.
Diversity of Spirotrichea.Colpodea, Nassophorea, Phyllopharyngea, Prostomatea and Plagiopylea
The next five classes, Colpodea, Nassophorea, Phyllopharyngea, Prostomatea and Plagiopylea, each contain from a few dozen to a few hundred species (Figure 303). The characters shared within each of the classes, which were formed most often by molecular phylogenies, are few in number and essentially ultrastructural. Colpodea are known for their ability to form cysts, allowing them to invade environments that frequently dry out. Sorogena stoianovitchae, a species that evolved an aggregative multicellularity (Figure 304) belongs to this class. Plagiopylea are microaerophilic or anaerobic and like Armophorea have hydrogenosomes. They inhabit the same biotopes as the Armophorea. Certain Phyllopharyngea, the members of the Suctoria subclass, have very particular morphologies, being completely devoid of cilia during the trophic phase (Figure 305); the other members of the class have more canonical morphologies and live free or in companionship of invertebrate animals. These fascinating Ciliophora live attached to many aquatic animals, and differentiate a ciliated form to move (Figure 305).
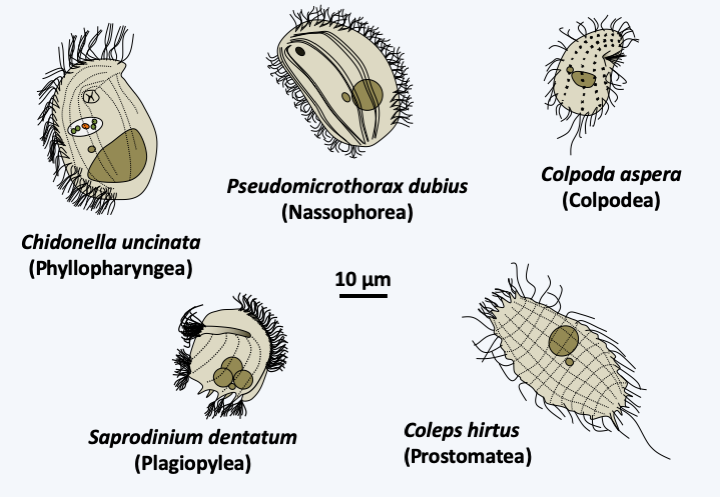
Figure 303.
Diversity of Colpodea, Nassophorea, Phyllopharyngea, Prostomatea and Plagiopylea.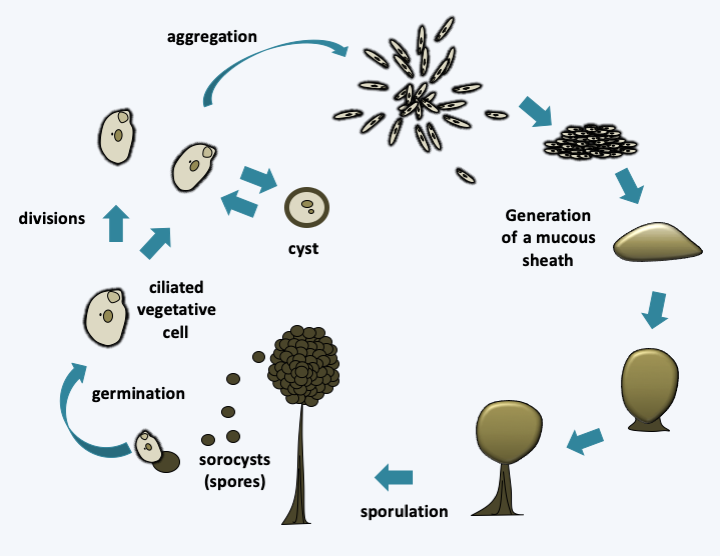
Figure 304.
Sorogena stoianovitchae cycle. During a deficiency, ciliated cells decrease in size and length. They aggregate and secrete mucus. They transform into sprorocysts while rising to the top of a small pedestal. Ciliated cells also have the ability to differentiate into cysts.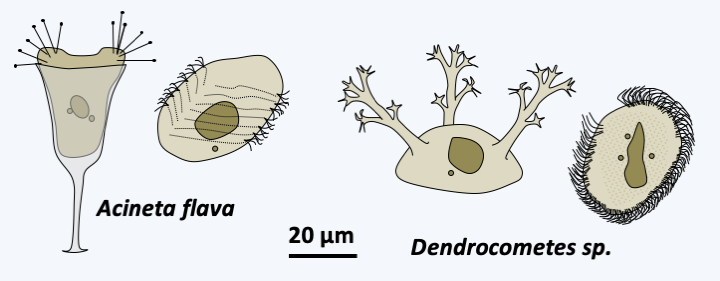
Figure 305.
Dimorphism of Suctoria. On the left, the un-ciliated trophic form and on the right the ciliated dispersal form.Oligohymenophorea
The last class of Ciliophora, Oligohymenophorea, is the most important in number of species, numbering in the thousands. The members present a diversity of forms (Figure 306) and functions with free species, marine or freshwater, species attached to the substrate in isolation such as Vorticella or grouped in single colonies such as Zoothamnium, parasites of mussels, such as Ophryoglena hemophaga, or fish, such as Ichthyophthirius multifiliis, etc. Sizes vary from 10 μm to over three millimeters! Many species change shape if nutrients are deficient, or for parasitic species when it is time to disperse. The name of Oligohymenophorea comes from the low number of cilia present around the cytostome (cell mouth), a character widely shared within the class. Many known species of ciliates belong to this group, such as Vorticella or the laboratory models Paramecium tetraurelia and Tetrahymena thermophila.
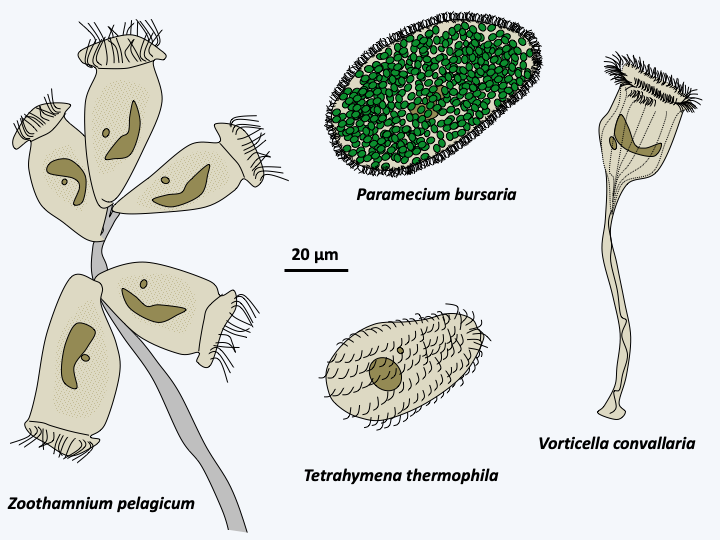
Figure 306.
Diversity of Oligohymenophora.Colponemidia & Acavomonidia
The rest of the known Alveolata appear to form a monophyletic group. However, the phylogenetic positions of Colponemidia and Acavomonidia as shown inFigure 295are not completely assured. These two branches have only a few species each. Colponemidia are small phagotrophic flagellates that present a ventral groove through which they feed (Figure 307). These organisms therefore retain the same “primitive” characteristics as those of Sulcozoa and Excavata, confirming their basal position in the Alveolata tree. Acavomonidia are also phagotrophic flagellates, but lack the ventral groove (Figure 307). As with Ciliophora, no evidence of the presence of a plastid during the evolutionary history of these two organism groups could be found. They are not capable of myzocytosis nor do they have the apical structure that participates in this process, unlike Myzozoa, the collection of Apicomplexa, Dinoflagellata and related minor groups (Figure 295). Likewise, the mitochondrial genome of Colponemidia and Acavomonidia does not exhibit the extreme simplification present in Myzozoa.
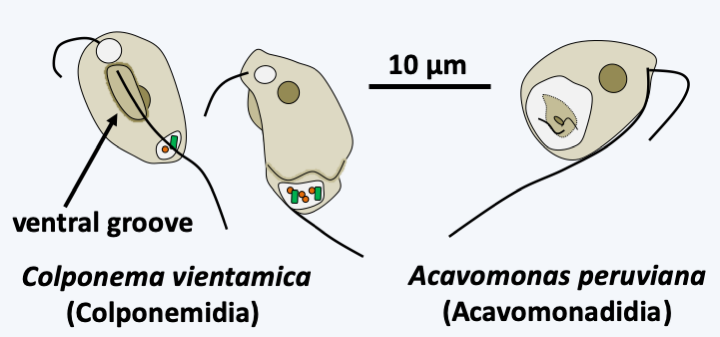
Figure 307.
Colponemidia and Acavomonidia.Myzozoa
The Myzozoa super-phylum therefore groups together two main groups, the Dinoflagellata and the Apicomplexa, which have strongly diverged from the ancestral type. It seems that their ancestor acquired the ability to cause myzocytosis through the acquisition of an apical complex (Figure 308) that resembles the cytopharynx of Katablepharida (Figure 248). This complex was then used in Apicomplexa to enter cells, making this group intracellular parasites of other eukaryotes. In Dinoflagellata, it would have been used before regressing to repeatedly change the plastid. Indeed, most Myzozoa have plastids. That of Apicomplexa has lost its photosynthetic capacity, but remains functional in certain related algae species, the Chromerida.
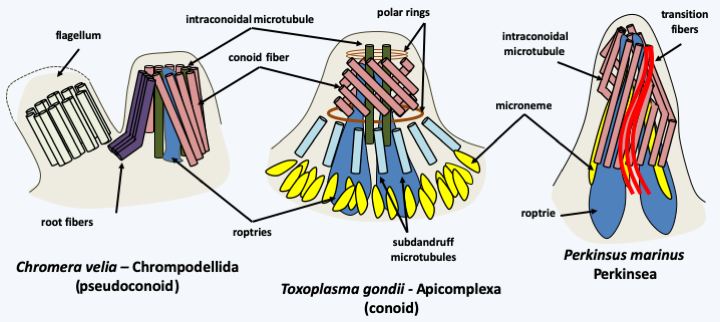
Figure 308.
Apical apparatus in three Myzozoa.In Apicomplexa and Chromerida, the plastid is surrounded by four membranes, an argument put forward to support a common origin to plastids in Hacrobia, Stramenopila and Alveolata. In contrast, in Dinoflagellata, the plastids have various origins (see pages 67-69) and are surrounded by two, three or five membranes! However, none is surrounded by four membranes, which calls into question the presence of a plastid having a unique origin in the Myzozoa… Another shared characteristic of these organisms is the extreme simplification of the coding capacity of the mitochondrial genome of the Myzozoa: it only codes for three proteins, cytochrome b and cytochrome oxidase subunits 1 and 3, and the two ribosomal RNAs.
Chrompodellida
The first monophyletic line of Myzozoa includes Chrompodellida and Apicomplexa (Figure 295). Chrompodellida form a monophyletic line of small phagotrophic flagellates as well as two algae possessing a plastid surrounded by four membranes and containing chlorophyll a, violaxanthin and β carotenes (Figure 309). Transcriptomic analyzes in a phagotrophic species, Voromonas pontica, revealed the presence of transcripts of genes functioning in the plastids, indicating that the non-photosynthetic species have probably used to have plastids but lost them. Molecular phylogenies suggest recurrent plastid loss within the group. Both algae in the group are endosymbionts of corals and have a trophic form without flagellum and a biflagellate zoosporic form; Vitrella brassicaformis is also able to differentiate autospores enclosed in a sporangium (Figure 309). Phagotrophic species like Colpodella feed on other protozoa through myzocytosis using their apical apparatus, which is called pseudoconoid because it does not form a complete cone. They have the ability to differentiate cysts. One of the algae, Chromera velia, has a pseudoconoid, while the other, Vitrella brassicaformis, appears to lack it. Chromera velia is, however, not known to be capable of phagocytosis. Both species are endosymbionts of corals.
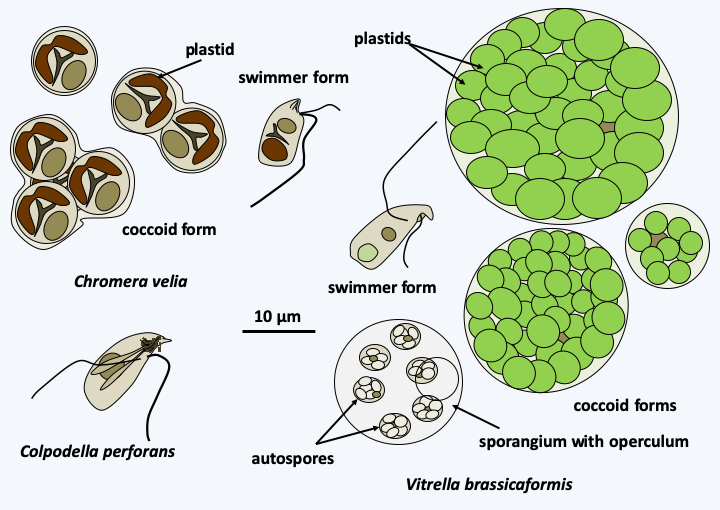
Figure 309.
Diversity of Chrompodellida.Apicomplexa
The Apicomplexa phylum is a group of about 5,000 species all of which are intracellular parasites of other eukaryotes. However, estimates suggest that this is the group of eukaryotes for which the number of species remaining to be described is greatest, as there are estimates of more than 5 million species. It is in this group of organisms that the most devastating human parasites are found (Table 13), such as Plasmodium, responsible for malaria (Box 22), Toxoplasma gondii causing toxoplasmosis, and Cryptosporidium, responsible for cryptosporidiosis in AIDS patients. Other animals are not better off, with coccidia such as Emeria and Theileria which decimates herds in Africa, Babesia which infect cattle and chickens or gregarines affecting insects.
Apicomplexa are characterized mainly by the presence of a complex apical apparatus composed of microtubules and specialized organelles, giving them their name (Figure 308). The mechanism serves for attachment and penetration of Apicomplexan cells into the host cell. The apparatus is associated at least for part of the life cycle with a conoid: the tubulin-based structure that appears to be the trace of the mechanism that was involved in myzocytosis in Apicomplexan ancestors. Apicomplexa have no apparent locomotion devices for most of their cycle, but are nonetheless capable of rapid movements. However, the male microgametes in Plasmodium have a flagellum which provides them with mobility towards the female gamete. Most species have a remnant of a plastid surrounded by four membranes, called an apicoplast. However, the Cryptosporidium seem to have lost it completely and have no trace of it even in their nuclear genome. The Cryptosporidium have also completely lost their mitochondrial genome and only have one mitosome; the other Apicomplexa have the mitochondrial genome typical of Myzozoa encoding three proteins and two ribosomal RNAs.
The phylogeny of these organisms is far from completed, especially as the majority of species remain to be discovered, and gene sequence data has made many changes to the traditional classification (Figure 310). Classically, the Apicomplexa were split into two classes: Aconoidasida and Conoidasida.
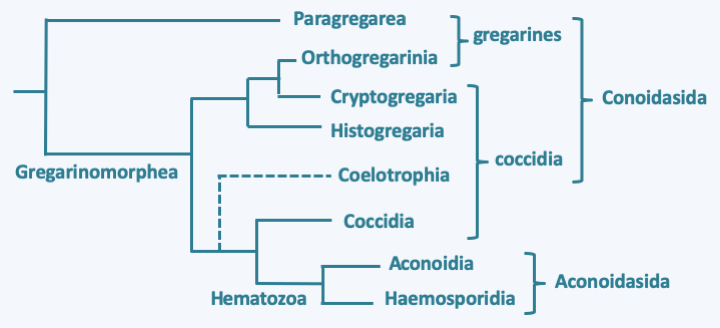
Figure 310.
Phylogeny of Apicomplexa.Aconoidasida are characterized, as the name suggests, by the fact that the conoid is present only at certain stages of the life cycle, whereas it is present at most stages in Conoidasida. This latter class appears to be paraphyletic with the Aconoidasida probably forming a monophyletic line from within it. The traditional ex-subclasses Coccidia and Gregarinasina, coccidia and gregarines in everyday speech, are most likely polyphyletic. Gregarines are large extracellular parasites inhabiting the internal cavities of invertebrates: intestines, coelomas, reproductive systems, etc. Nearly 2,000 species have been described. Traditionally, they were divided into three orders: Archigregarinorida infecting marine invertebrates, Neogregarinorida attacking only terrestrial invertebrates and Eugregarinorida, the most numerous, having a broad spectrum and parasitizing marine, freshwater or terrestrial invertebrates. Molecular phylogenies show that the last two orders are probably not monophyletic andFigure 310proposes their division into two lineages: Paragregarea and Orthogregarinia. Paragregarea diverge at the base of the Apicomplexa evolutionary tree. All gregarines are characterized by the presence of a head-like apical apparatus at the anterior pole of the cell. The apparatus is called epimerite if it is separated from the rest of the cell body by a septum, delimiting a cell compartment without nucleus, and mucron if it is continuous with the rest of the cell body (Figure 311andFigure 312). This “head” is always present in Archigregarinorida, in which it allows attachment to the host and participates in nutrition through myzocytosis (Figure 313), but is only present during the sporozoite stage in other gregarines. Some species, particularly those classified as “ex” archigregarines, appear to have completely lost the plastid, while a vestige of it is present in other gregarines. Most species have a sexual cycle (Figure 314) and a few species have the possibility of asexual multiplication by schizogony. They only need one host to complete their life cycle. Coccidia are small intracellular parasites that infect animals; several thousand species have been described. Some of these organisms have now been separated into independent subclasses, restricting Coccidia to a defined set of parasites related to Aconoidasida (Figure 310).
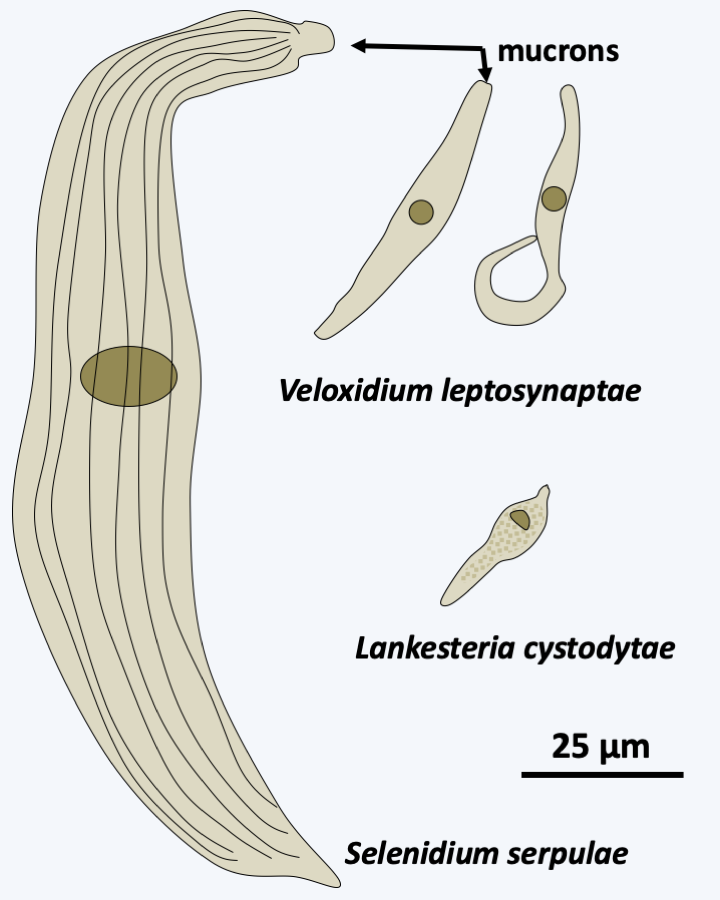
Figure 311.
Diversity of Paragregarea. Selenidium serpulae parasitizes the annelid Serpula vermicularis, Veloxidium leptosynaptae parasitizes the sea cucumber Leptosynapta clarki, Lankesteria cystodytae infects the sea squirt Cystodytes lobatus. </figcaption> </figure>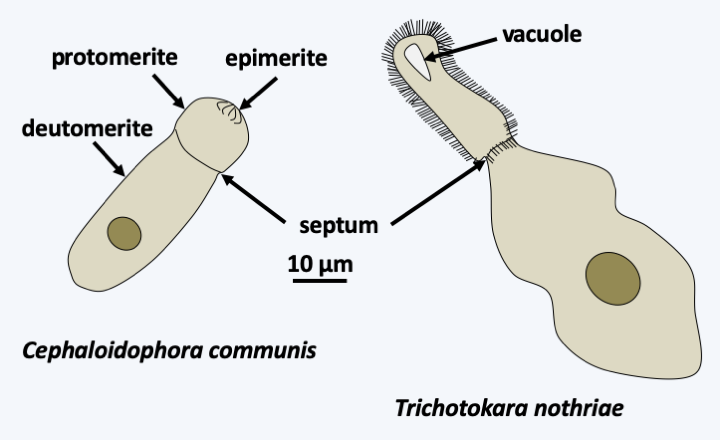
Figure 312.
Diversity of Orthogregarea. Cephaloidophora communis parasitizes barnacles of the genus Balanus and Trichotokara nothriae the annelid Nothria conchylega. The extensions on the protomerite of Trichotokara nothriae are not flagella.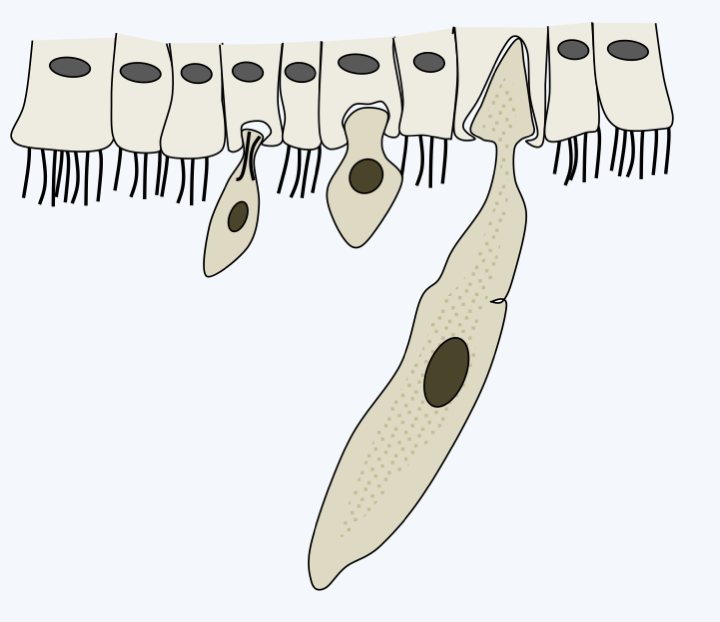
Figure 313.
Typical development of gregarine trophozoites. After attachment to host cells, gregarines pump nutrients, often by myzocytosis in 'archigregarines', through the membrane in other species.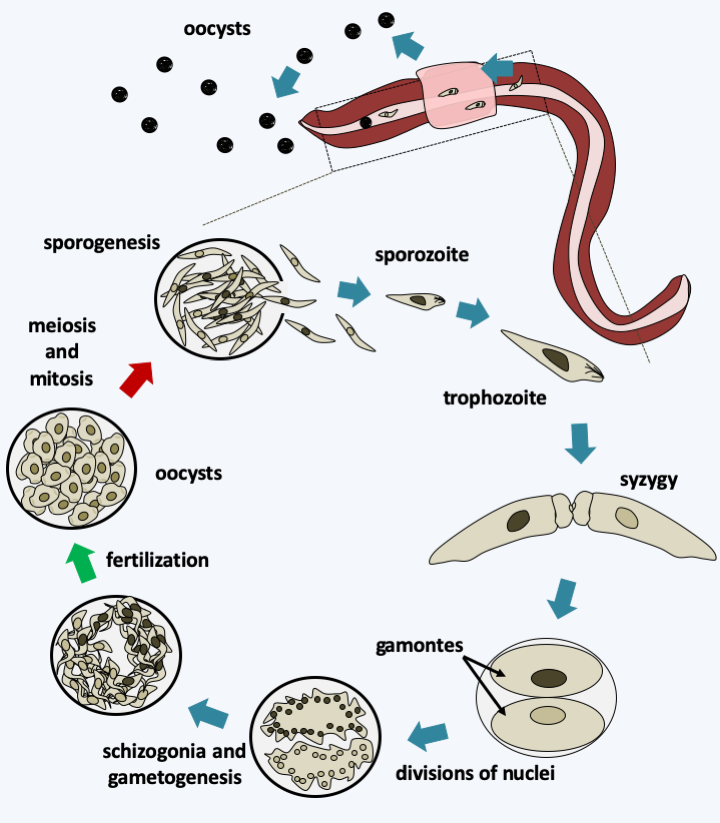
Figure 314.
Life cycle of the gregarine Monocystis agilis. This species lives in the seminal vesicles of earthworms. The cycle begins with the ingestion of an oocyst by an earthworm and the release of the sporozoites. The motile sporozoites cross the intestinal barrier and will colonize the seminal vesicles, where the sperm differentiate, passing through the blood network. In the vesicles, the sporozoites transform into trophozoites which greatly increase their size by feeding on the spermatocytes of their host. When they are mature, they pair up in a process called syzygy which is the prelude to sexual reproduction. Syzygy is followed by encystment of the two cells to form two gamonts and nuclear divisions without cytokinesis. The two cells end up forming a schizogony - which is called merogony in the Apicomplexa. Fertilization takes place in the oocyst and gives rise to zygotes which immediately undergo meiosis. Meiosis is followed by mitosis and differentiation of haploid cells into sporozoites. Mature oocysts exit the worm through the genital pore and wait to be ingested by a new worm. It is also possible that they are transmitted during mating.### Paragregarea The most recent data therefore suggest a division of Apicomplexa into two classes: Paragregarea and [Gregarinomorphea](#gregarinomorphea). The first contains only gregarines, while the second contains gregarines, coccidia and [Aconoidasida](#aconoidasida). The Paragregarea (Figure 311) group together most of the ancient Archigregarinorida and some Eugregarinorida. These organisms live in the intestine or the coelomic cavity of marine (molluscs, annelids, ribbon worms, echinoderms, etc.) or terrestrial (millipedes) invertebrates.
### Gregarinomorphea #### Orthogregarinia Gregarines belonging to Gregarinomorphea are grouped within the subclass of Orthogregarinia (Figure 312). They have varied hosts; marine, freshwater or terrestrial. They share morphology and function with other gregarines as described above.
#### Cryptogregaria The Cryptogregaria subclass currently contains only one genus, _Cryptosporidium_. These organisms are parasites of the intestines of terrestrial vertebrates such as amphibians, birds or mammals. _Cryptosporidium parvum_ and _Cryptosporidium hominis_ are common parasites that infect humans (Table 13). While _Cryptosporidium hominis_ is exclusive to humans, _Cryptosporidium parvum_ is capable of infecting other mammals. Both species cause diarrhea in healthy humans. In immunocompromised individuals, they persist and may lead to death. These parasites have undergone a very important reduction evolution because their mitochondria have evolved into mitosomes and their plastids have completely disappeared. _Cryptosporidium_ have long been classified with coccidia because it seemed that they had a particular intracellular phase called "epiplasmic": the parasite remained under the plasma membrane without entering the cytoplasm. This localization is no longer considered to be correct, because it seems that the _Cryptosporidium_ are able to differentiate a particular membrane structure which protects the cell and takes the appearance of a parasitophorous vacuole when the cell is affixed to that of its host. (Figure 315). In accordance with this proposed parasite structure, molecular phylogenies clearly place Cryptogregaria with gregarines, predominantly extracellular parasites. The life cycle of _Cryptosporidium_ is very similar to that of gregarines (Figure 316). After infection of a host, they differentiate into cysts, which are infectious propagules, that may be ingested by subsequent hosts with the food if it becomes contaminated with the cysts. These cysts are very resistant and can persist, for example, in swimming pools treated with bleach.
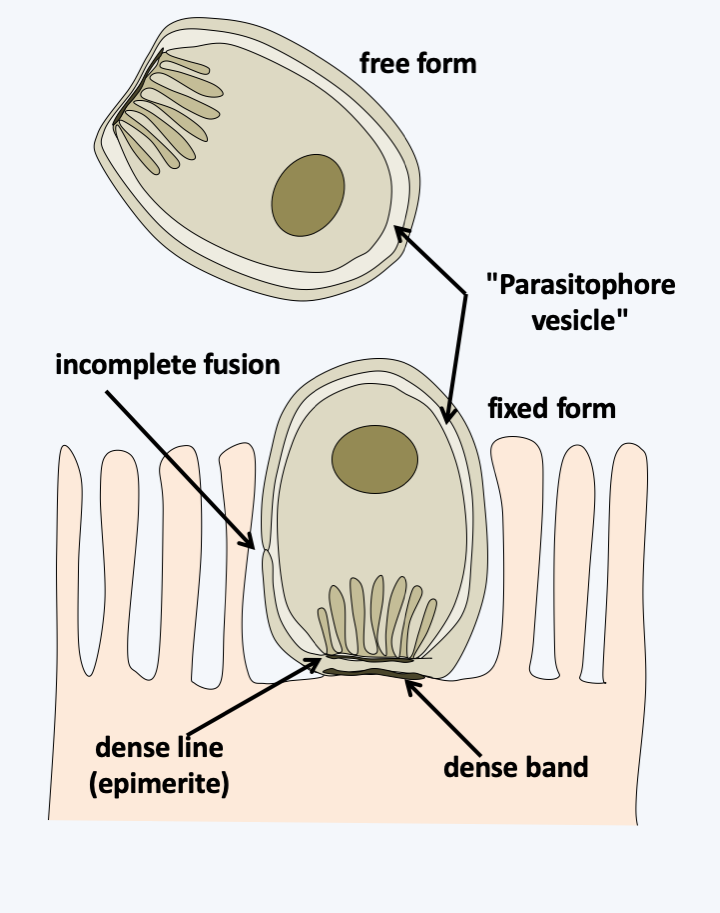
Figure 315.
Structure of Cryptosporidium trophozoites. Trophozoites are nested in a 'parasitophore vesicle' that has long been thought to derive from the host cell. Discovery of the same structure in free forms found in biofilms calls into question the origin of the vesicle. Indeed, the structure is compatible with a derivation from the Cryptosporidium membrane and fusion, as observed in Labyrinthula.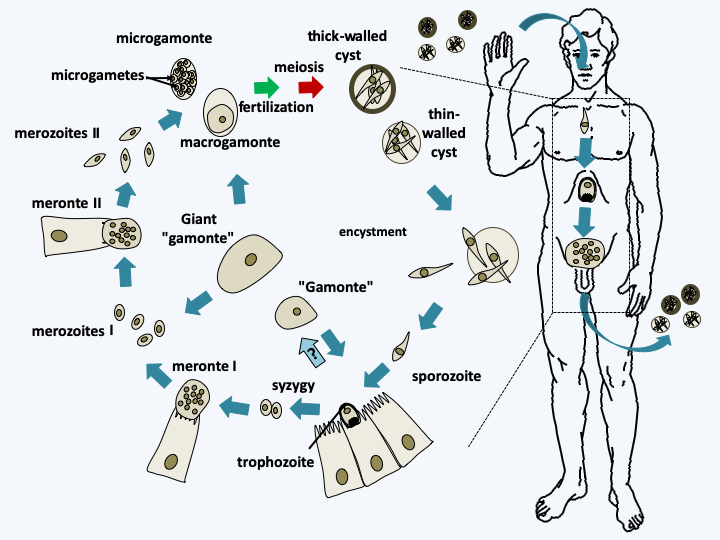
Figure 316.
Cryptosporidium cycle. The cycle begins with the ingestion of cysts containing four cells from meiosis. Their disencystation releases the sporozoites which transform into trophozoites which may or may not attach to cells in the intestine. These join together in pairs and form a syzygy. They infect new cells to give rise to the first so-called type I meronts which transform after schizogony into type I merozoites. These in turn infect new cells and transform into type II meronts, then after schizogony of new in type II merozoites. The latter infect new cells and transform into either macrogamonts or microgamonts containing 16 spermatozoa. Fertilization occurs and the zygote turns into a cyst in which meiosis takes place. Cysts have two types of lining. For 80% of them the wall is thick and for 20% it is thin. These latter cysts can probably hatch in the patient's intestine, causing self-infection. In addition to these different stages, Cryptosporidium can differentiate into giant cells known as gamonts and giant gamonts outside the cells. Their origin is not clear, but they probably originate from trophozoites.#### Histogregarida The Histogregarida subclass contains only a few species which parasitize marine invertebrates. Like the [Cryptogregaria](#cryptogregaria), these organisms were previously placed either with gregarines or with coccidia. Molecular phylogenies now clearly show that they are related to [Cryptogregaria](#cryptogregaria) and [Orthogregarinia](#orthogregarinia). They are characterized by the absence of schizogony after syzygia (Figure 317). Their trophozoites, the cells involved in absorbing nutrients, are nestled between their host's cells and lack mobility.
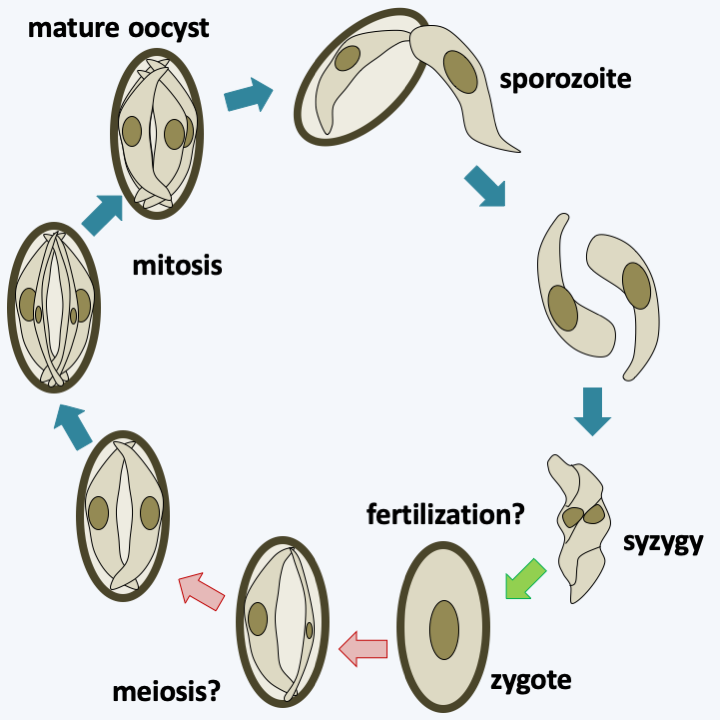
Figure 317.
Putative cycle of Gemmocystis cylindrus. This Histogregarida parasitizes the coral Dendrogyra cylindrus. Oocysts usually containing six sporozoites hatch and give rise to free sporozoites. These enter the gastric cells or remain nested between them. Two sporozoites associate in a stage that appears to be syzygy. The two cells appear to fuse together in what is likely fertilization and then divide asymmetrically in the oocyst to give rise to six sporozoites. Meiosis has not been demonstrated and may occur in an alternate host. Mature oocysts appear to be discharged into the gastric cavity of the coral.#### Coelotrophia The Coelotrophia subclass has been established for Apicomplexans that have an intracellular phase followed by an extracellular phase in the polychaete worm coelom. Coelotrophian gametes have a particular morphology (Figure 318). The absence of sequence data makes it difficult to position these organisms with certainty on the Apicomplexa tree (Figure 310).
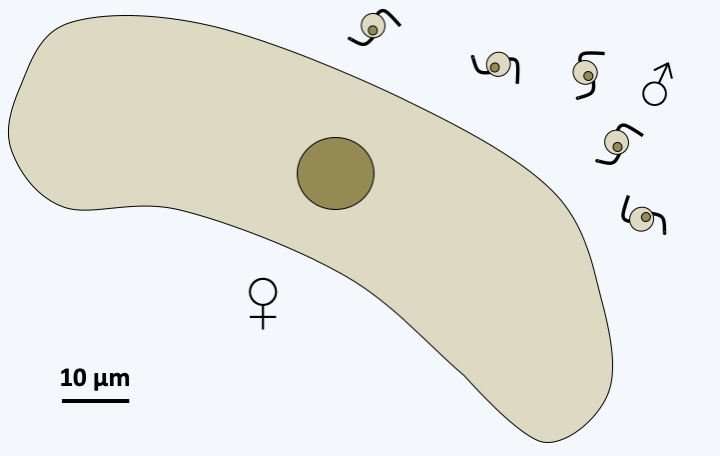
Figure 318.
Gametes of Coelotropha durchoni.#### Coccidia The Coccidia subclass, several thousand species strong, contains obligate intracellular parasites of vertebrate and invertebrate animals. Currently, these coccidia are provisionally classified in three orders, Adeleida, Eimeriida and Ixorheida. Some complete their cycle with a single host, while others, traditionally placed in the order of Adeleida, alternate between a tick and a vertebrate. Note that part of the Adeleida completes their entire cycle in an invertebrate, suggesting that species alternating between two hosts have acquired the ability to invade their vertebrate hosts secondarily. This is not the case for all Coccidia, as species of the order Eimeriida only infect vertebrates. The Coccidia that diverge first from the rest infect fish, and molecular phylogenies indicate that there are at least two suborders whose phylogenies roughly follow those of their hosts. It therefore seems that there were two separate "host/parasite" co-evolution phenomena within this order. Like the gregarines, the Adeleida make a syzygy as a prelude to sexual reproduction, while in the other Coccidia, fertilization results from the fusion of separately produced gametes. The best known species is _Toxoplasma gondii_ which causes toxoplasmosis (Table 13). This parasite, belonging to the order Eimeriida, has a very broad host spectrum and infects all species of mammals. However, it is established that the cat is the definitive host where the sexual phase occurs (Figure 319). Other known species are _Isospora belli_ and _Cyclospora cayetanensis_ affecting humans (Table 13) as well as _Neospora_ which infects dogs and cattle.
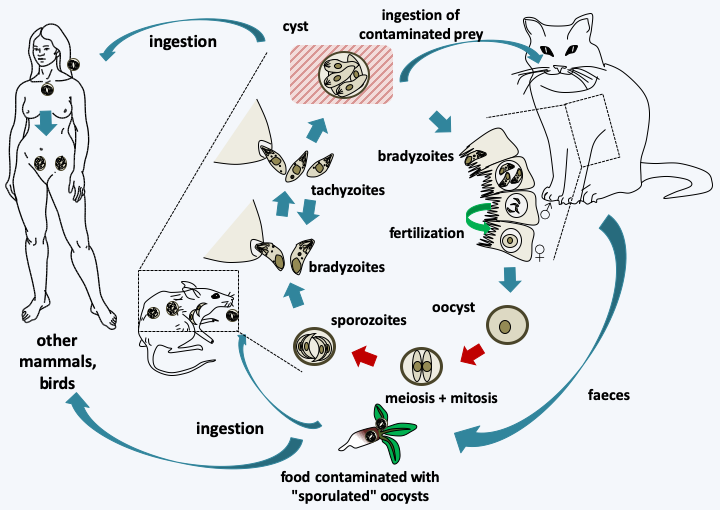
Figure 319.
Life cycle of Toxoplasma gondii. This species requires two hosts to complete its cycle, the cat which is the host in which sexual reproduction takes place and another warm-blooded animal serving as an intermediate host such as a mouse or bird, in which asexual reproduction takes place. The intermediate host becomes infected by ingestion of food contaminated with sporulated oocysts, that is, in which eight sporozoites are differentiated after meiosis and mitosis. Desencystment leads to slowly dividing bradyzoites which infect cells of the host. These subsequently differentiate into rapidly dividing tachyzoites, and then into cysts that will persist in the host's tissues, usually muscles or brain, for several months or years. When a cat consumes the prey, the encystment leads to bradyzoites which will invade the intestinal cells and divide there by adopting up to five successive forms reproducing by binary fission or schizogony. At the end of these cycles of asexual divisions, male microgamonts and female macrogamonts are differentiated. Fertilization leads to the production of oocysts which will be evacuated with the feces of the cat. These continue to mature in the environment. Other mammals or birds become infected by consuming food infected with oocysts or cysts. Toxoplasma gondii is capable of infecting a large number of species of mammals and birds, including humans. Transmission during gestation of the parasite to the fetus usually leads to its death.#### Hematozoa The subclass of Hematozoa covers the old class of Aconoidasida. It is subdivided into two "super orders". The first, that of Aconoidia, contains the orders Piroplasmida and Nephromycida. The former are blood parasites of birds and mammals and are transmitted by ticks (Figure 320). The genera _Babesia_ and _Theileria_ are the most species rich. These two genera, based on morphological and serological data, are polyphyletic and their reclassification is under development. Piroplasmidae have a major impact on livestock, but recently babesiosis is also emerging in humans (Table 13). The order Nephromycida contains a single genus, _Nephropmyces_, which appears to encompass the only mutualistic species of Apicomplexa. Indeed, these organisms, for a time classified as fungi, hence their name, systematically inhabit the cavities of a particular organ of ascidians (tunicates and sea squirts) of the genera _Molgula_ and _Bostrichobranchus_, the "renal sac". The presence of _Nephropmyces_ in all ascidians is all the more remarkable as young ascidians must acquire their _Nephropmyces_ from the environment as they are not transmitted vertically. It is therefore suggested that they are not parasites, but at least commensals, probably mutualists whose role is not clearly understood. _Nephropmyces_ appear to harbor endosymbiotic bacteria which have the ability to break down urate, whereas the sea squirt cannot. _Nephropmyces_ would therefore participate in the prevention of uric acid "stones" in these Urochordata! In addition to their special relationship to their host, these organisms are extracellular, unlike all other Hematozoa and the [Coccidia](#coccidia) to which they are related. Nephromycida differentiate several forms, including a biflagellate form with the two flagella positioned at the back of the cell (Figure 321).
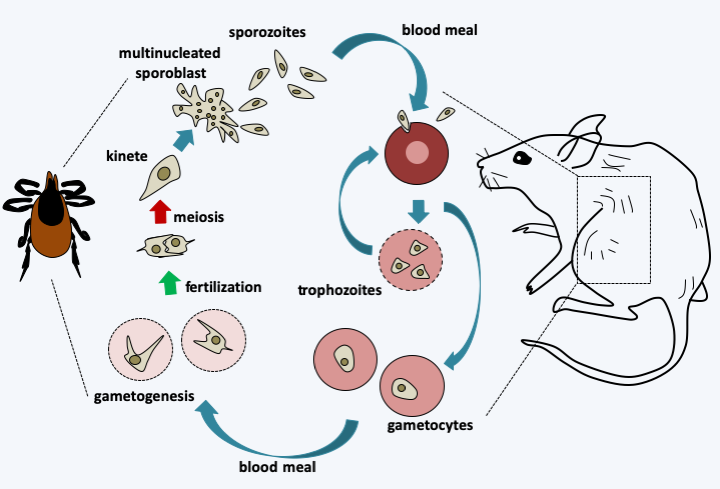
Figure 320.
Babesia microti life cycle. This species primarily attacks mice but, like many other Babesia species, can occasionally infect humans and even be transmitted from person to person through blood transfusions. The cycle begins when an infected tick eats a blood meal from its host. Sporozoites are injected with saliva and enter the interior of red blood cells where they divide by binary fission. The trophozoites produced can infect other erythrocytes or differentiate into gametocytes. During a blood meal, the tick becomes infected and the gametocytes differentiate into gametes in its intestine. Fertilization occurs and is immediately followed by meiosis, producing kinetes that migrate to the salivary glands. These differentiate into plurinucleated sporoblasts which generate sporozoites. These will be transmitted during a new blood meal by the tick.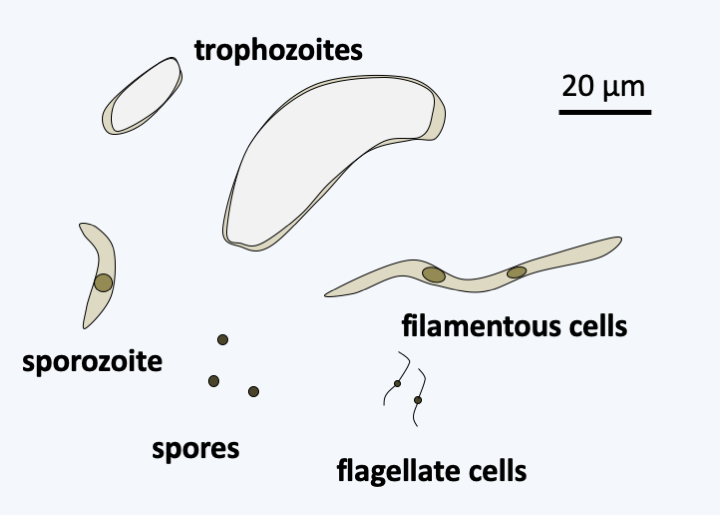
Figure 321.
Nephromyces retortiformis.#### Haemosporidia The Haemosporidia superorder contains several hundred parasites of terrestrial vertebrates: reptiles, birds or mammals. They are transmitted by blood-sucking Diptera (flies and mosquitoes), in which they reproduce sexually. When these vectors bite a vertebrate host, the parasites infect epithelial and / or liver cells. The released parasites then infect other tissues where they continue to develop. They eventually infect erythrocytes, before being taken by the Diptera vectors during their blood meal. The best known species belong to the genus _Plasmodium_. In humans, _Plasmodium_ cause malaria (Box 22).
### Dinozoa The last known line of Alveolata is that of Dinozoa (Figure 295). It is currently subdivided into three groups of unequal importance. The Perkinsea class contains a few intracellular parasitic species of tadpoles, molluscs or other protozoa. The best-known species is _Perkinsus marinus_, which attacks oysters, devastating oysters farms (Figure 322). More recently, a line of Perkinsea has been associated with massive tadpole mortalities. These organisms have an apical complex, the function of which is not yet understood, but is probably involved in nutrition. Genome analyzes and ultrastructural data suggest the presence of a relic of a plastid, indicating that these organisms likely underwent simplifying evolution from a photosynthetic precursor. The Ellobiopsea class also contains only a few species that parasitize crustaceans. They have a structure which superficially resembles [Chrytridiomycota](/ebook/book/Part2/phylogenetic_classification/sections/Ascomycota.html#chrytridiomycota) fungi (Figure 323). Ellobiopsea disperse using zoospores, but the structure of the flagellar apparatus is not yet known.
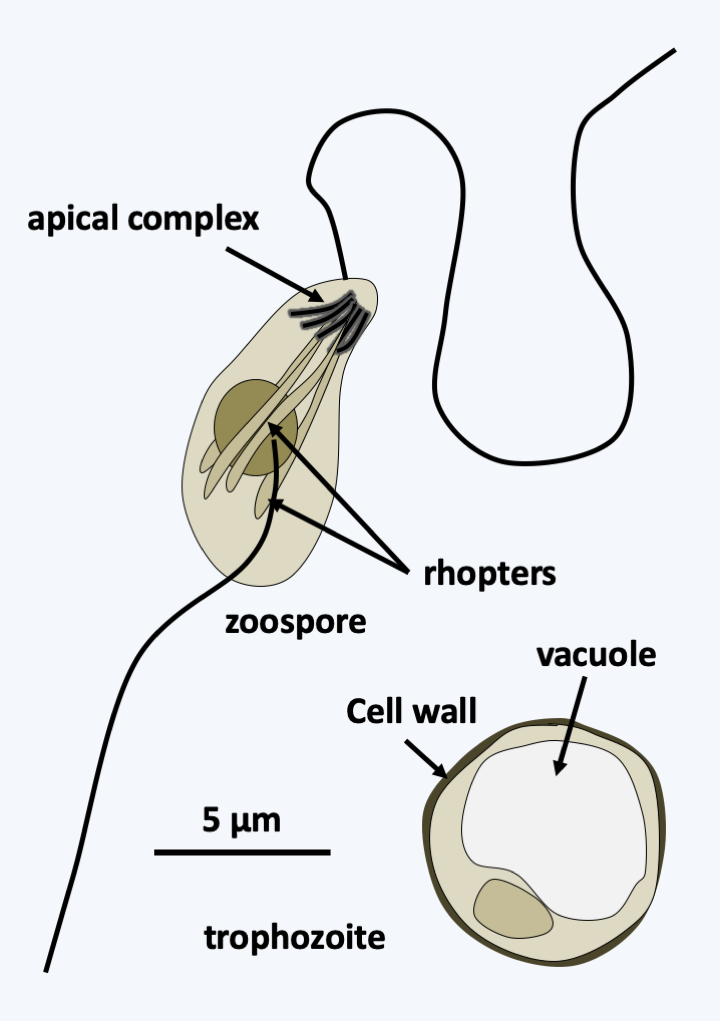
Figure 322.
Perkinsus marinus.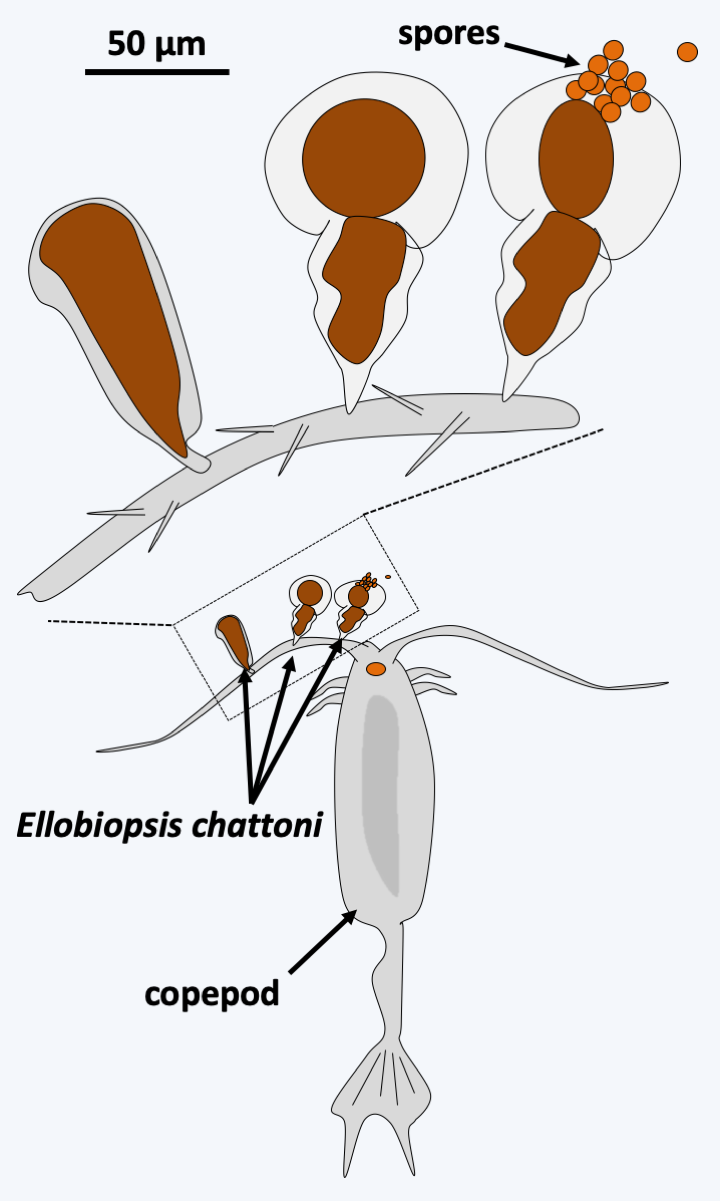
Figure 323.
Ellobiopsis chattoni.#### Dinoflagellata With several thousand described species, the branch of Dinoflagellata constitutes the major lineage of Dinozoa (Figure 295). These protists have adopted many lifestyles. Half of them are photosynthetic, other phagotrophs, a few appear to be osmotrophic, and several are parasitic, or mutualistic, symbionts like zooxanthellae. Many are mixotrophic and exhibit great nutritional plasticity, as they can either live off photosynthesis, phagotrophy, or osmotrophy, depending on the conditions they encounter. The free planktonic species are marine or in freshwater; there are even some species that live specifically in melting snow. Dinoflagellata are characterized mainly by distinctive nuclear structures, associated with particular modalities of mitosis ([Figure 14](/ebook/book/Part1/intermediary/sections/eukaryotes_without_organelles.html#dinos-chromo) and Box 23). These structures result from the replacement of histones in chromatin by other basic proteins resembling the "histone-like" proteins of prokaryotes. Histone genes are still present in the genomes of these organisms, but the histones they encode are widely divergent from the histones of other eukaryotes. In addition, they are transcribed only to a low level. Dinoflagellata also have two unequal flagella inserted laterally. Usually, one of the two flagella wraps around the cell and exhibits characteristic undulations, while the other is straight and faces forwards or backwards depending on the Dinoflagellata clades (Figure 324). Photosynthetic species have plastids of very different origins (Table 5). Most types of plastids are clearly the result of recent replacements linked to tertiary endosymbiosis (see page 67-69). However, the origin of the plastid in Dinoflagellata is still a big mystery. Indeed, the plastid that seems the most widespread, and is therefore generally assumed to be ancestral, is surrounded by three membranes and contains peridinin, unlike the plastid of Apicomplexa which is surrounded by four membranes. However, it is possible that this peridinin plastid is also the result of tertiary endosymbiosis. The discovery of a plastid relic among the early-diverging groups _Psammosa_ or Oxyrrhinales would therefore be very interesting. Indeed, these early-diverging lineages contain phagotrophs (Figure 325). The discovery in these species of a plastid relic and in particular the analysis of its structure could therefore make it possible to draw a conclusion, all the more so if the relic is surrounded by four membranes...
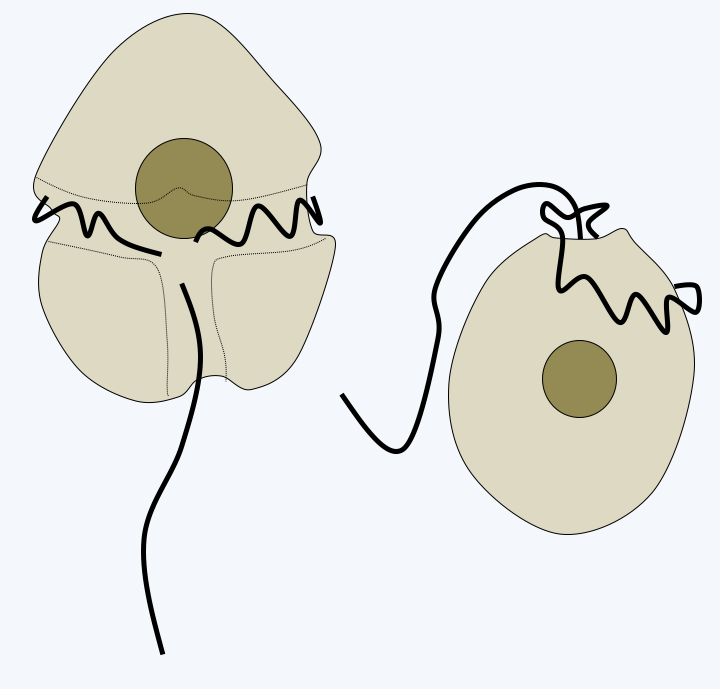
Figure 324.
Morphology of Dinoflagellata. On the left, typical structure with a lateral flagellum and a posterior flagellum. Right, structure in prorocentroids, a group whose monophyly is not yet definitively established. In this group, both flagella are inserted into an anterior pocket.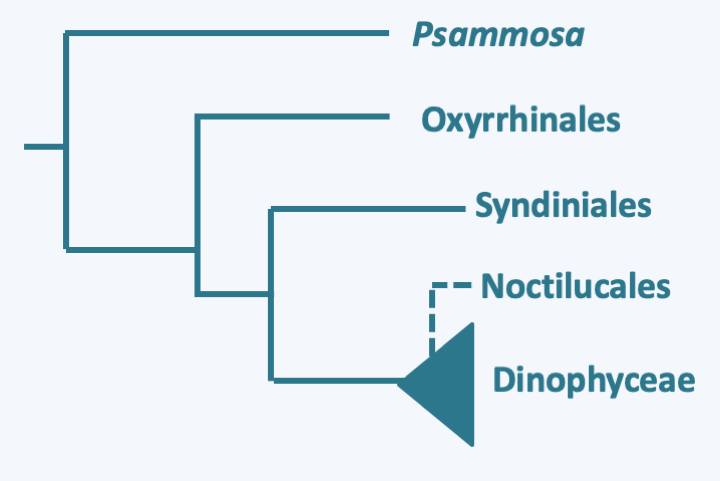
Figure 325.
Phylogeny of Dinoflagellata.Molecular phylogenies show that the genus _Psammosa_ is the one that diverges first in the Dinoflagellata evolutionary tree (Figure 325). These small biflagellate phagotrophs have an apical complex resembling that of _Perkinsea_ (Figure 326). Their nucleus, said to be of the syndin type (Box 23), contains chromosomes with condensed chromatin positioned at the periphery of the nucleus. The lineage which then diverges is that of the Oxyrrhinales which contains only one species: _Oxyrrhis marina_ (Figure 326). This species is used as a laboratory model to study the evolution of predation and phagotrophy. Devoid of apical complex, it feeds conventionally by phagocytizing a variety of prey including various protozoa, algae, fungi, bacteria or small animals, even members of its own species! But it can also feed by osmotrophy and use ethanol or acetate as a carbon source. Its growth is improved in the presence of light, probably thanks to the rhodopsin it has in its vacuoles, which is a result from the horizontal transfer of an eubacterial gene (see page 71). It also has a syndin-type nucleus and two flagella that carry mastigonemes. Its mitochondrial genome carries only two protein coding genes: cytochrome b and the cox3 subunit of cytochrome oxidase. Their expression is special because the two coding sequences fuse to become a single gene at the mRNA stage. The last basal line is that of Syndiniales. It contains a few dozen intracellular parasites of invertebrates, fish eggs or other protists including Dinoflagellata. Their simple life cycle involves a biflagellate cell for dispersal (Figure 327). Their nucleus presents the typical characteristics of the syndin nucleus, with a peripheral chromatin more or less condensed according to the stage of development. These organisms do not have an apical complex, but enter cells using a similar structure. The life cycles of _Psammosa_, Oxyrrhinales and Syndiniales are probably still incompletely known because sexual reproduction has not been described.
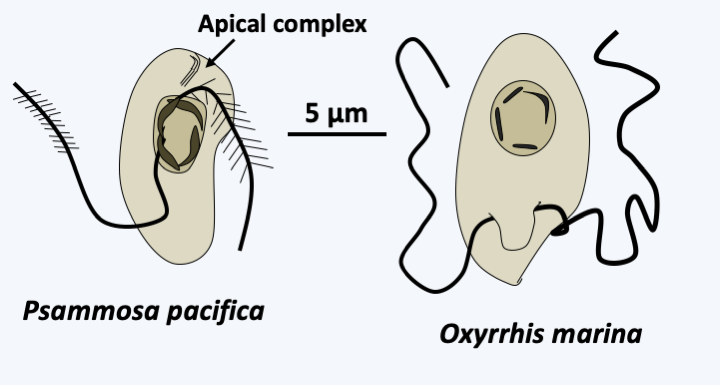
Figure 326.
Early-diverging dinoflagellata.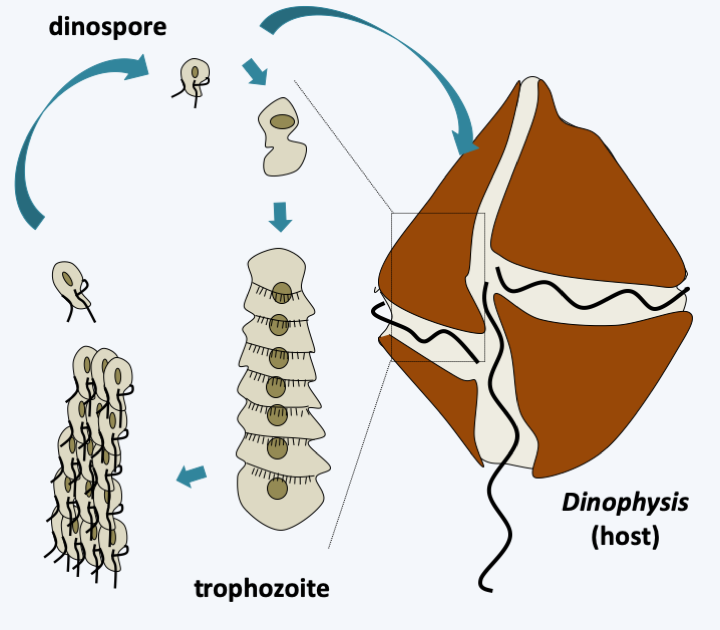
Figure 327.
Cycle of Amoebophrya sp. Dispersal occurs via biflagellate dinospores. They penetrate inside their host and travel to the nucleus where they differentiate into multinucleated trophozoites which eventually take on a worm form. After schziogonia, they transform into zoospores which emerge from their host after its lysis.The last lineage of Dinoflagellata, the class Dinophyceae, is the most important regarding number of species. It is characterized by the presence of two heterochontic flagella, enabling motility through swimming, and a dinokaryon (nucleus of dinoflagellates; Box 23). Note that in some parasitic species, the dinokaryon chromosomes can partially decondense. Most species have large, isolated cells; but a few rare species, such as _Dinotrhix paradoxa_, form simple colonies comprising a few cells (Figure 328). Some species have their cells empty or containing a little material which appears shapeless under an electron microscope, while others, forming a monophyletic group, have cells containing cellulose plaques (Figure 329). This structure, called the **amphiesma**, gives cells a complex shape (Figure 328). It can be reinforced by an additional layer located further inside the alveoli and composed of cellulose mixed with dinosporin, a substance similar to sporopollenin also involved in the formation of the wall of the cysts. This layer, called **pellicle** (= skin) can also be present in species without cellulosic plaque such as in Noctilucales. There is no typical apical complex, but in species capable of myzocytosis there is a structure associated with microtubules called a "peduncle". This structure involved in myzocytosis is probably a relic of the apical complex typical of [Myzozoa](#myzozoa). Species belonging to the order Noctilucales are well known and capable of bioluminescence. They use an enzyme for bioluminescence called luciferase, and a tetrapyrrolic substrate, luciferin. A few species have structures that can capture light. These are made up of lipid droplets stacked between membranes containing carotenes. In some species a structure focuses the light on these "eyes". Many activities of these organisms are regulated by light, such as photosynthesis and bioluminescence.
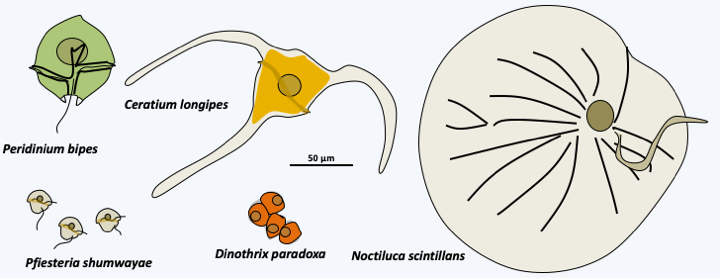
Figure 328.
Diversity of Dinophyceae.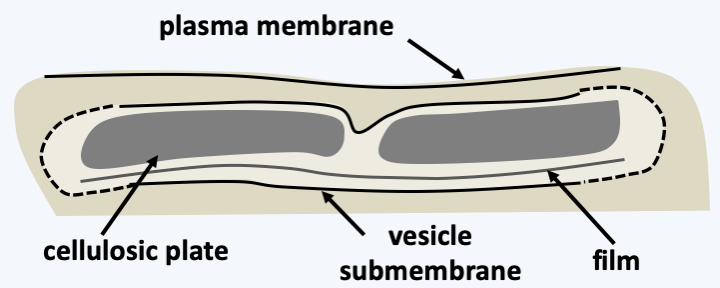
Figure 329.
Structure of the amphiesma.The most common plastid in Dinophyceae contains peridinin. The plastid genome has acquired a surprising structure ([Figure 42](/ebook/book/Part1/major_events/sections/plastids.html#dino-plsatid-genes)) and its mode of expression is also non-canonical because it involves messenger editing and the addition of polyuridine tails. In other types of plastids, the structure and expression most often resembles that of the cell from where the plastid originated. However, in _Karlodinium_, whose fucoxanthin plastids are derived from [Haptophyta](/ebook/book/Part2/phylogenetic_classification/sections/Hacrobia.html#haptophyta}), the structure and expression of the genome resemble that of peridinin plastids. The mitochondria of Dinophyceae are no less original because their genome codes for the same incomplete rRNAs and the same three proteins (cox1, cox3 and cob) as the mitochondrial genome of [Apicomplexa](#apicomplexa). However, it is made up of linear molecules encoding a single gene. They have varying sizes and stoichiometries. They are also riddled with non-coding sequences. Finally, some transcripts must be cross-spliced and/or edited to be functional. Sexual reproduction is known for a few species (Figure 330), but meiosis appears to be very different from classical meiosis. Meiosis does not appear to have been described cytologically, but genetic data obtained from _Crypthecodinium cohnii_ suggests that it consists of only one division or two divisions without recombination. Some Dinophyceae algae can differentiate cysts that remain dormant until suitable conditions are present (Figure 330).
Back to chapter index