Stramenopila
Back to main indexJump to section:
Introduction
The Stramenopila super-phylogeny, also informally called “heterokonts”, has been defined primarily by molecular phylogenies. Together with Alveolata and Rhizaria, it forms a monophyletic group known either by the acronym SAR (Stramenopiles + Alveolata + Rhizaria) or by the name Harosa. They bring together many organism groups that at a glance have little in common. Presumably, this is the group of eukaryotes in which evolution has experienced the greatest number of planes of unicellular and multicellular organization. It is also one of the most successful groups of eukaryotes, which has invaded practically all biotopes. Stramenopila proliferate in marine, freshwater and also terrestrial environments. They have explored many ways of living, since the super-branch contains phagotrophs, fungal-like organisms, algae, as well as parasitic or mutualistic symbionts. Currently known species represent only a small part of their diversity, as metagenomic analyzes have identified more than ten lineages about which nothing is known so far. The phylogeny in Figure 254 therefore only indicates lines for which species have been characterized other than by just metagenomic analyzes.
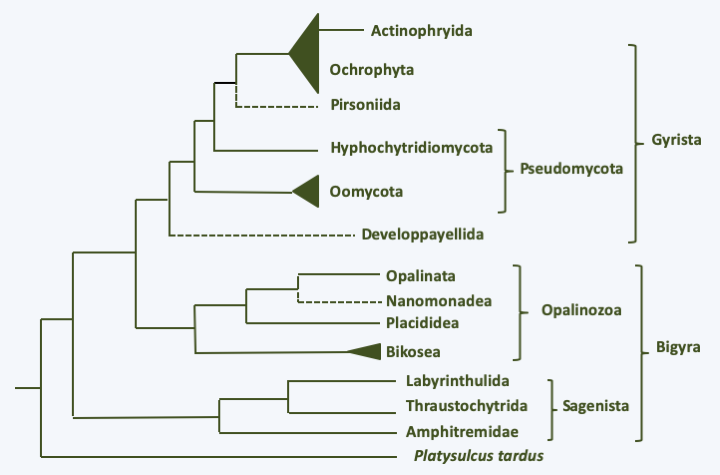
Figure 254.
Phylogeny of Stramenopiles.The Stramenopila are characterized for the most part by the presence at some point in their life cycle of a cell possessing two flagella, the most anterior of which carries mastigonemes in one or two rows (Figure 255). These consist of three parts of distinct diameters and are therefore called tripartite. Mastigonemes consists of proteins and originate from the Golgi apparatus. This heterochronous flagellar apparatus gave them their name. Species that do not have these two flagella form monophyletic subsets nested within groups with the typical two flagella, showing that these are secondary losses. All Stramenopila analyzed have tubular crested mitochondria. Some groups are protected by siliceous envelopes or skeletons called thecae (sing. theca), such as diatoms (Bacillariophyta) or Dictyochophyta. The accumulation of these siliceous structures forms an important part of sediments and is used by paleontologists for dating and studies of fossil biotopes. These various “theca” or envelopes make it possible to date the appearance of the Stramenopila to more than 700 million years in the past.
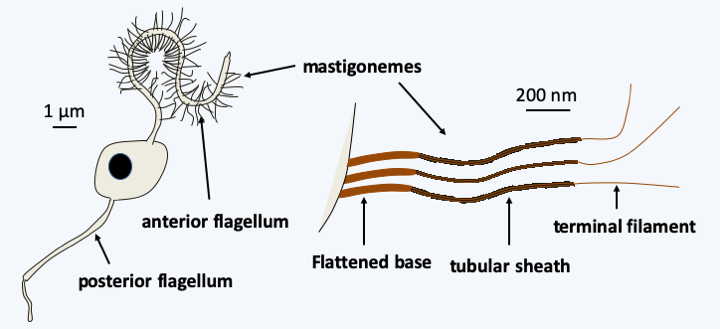
Figure 255.
Structure of flagella in typical stramenopile/heterokont.At the base of the evolutionary tree is the branch with Platysulcus tardus, a small flagellate possessing the flagellar apparatus typical of Stramenopila, except that the mastigonemes appear to be bipartite (Figure 256). The tree is then currently subdivided into three major branches, the Sagenista, the Opalinozoa and the Gyrista (Figure 254). In all three clades, there are phagotrophs which generally diverge rapidly from other members.
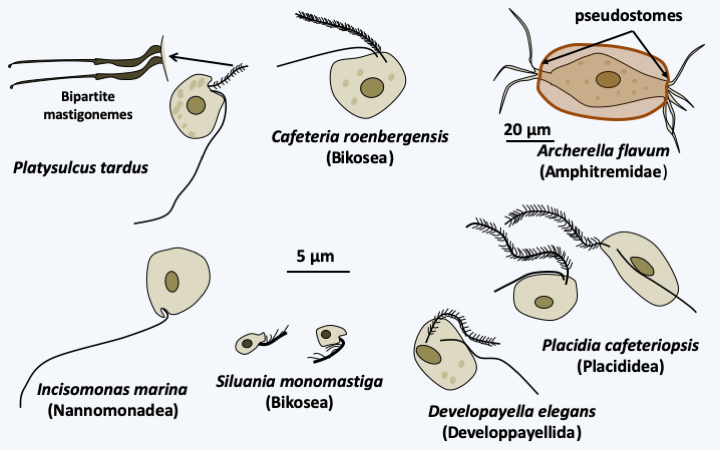
Figure 256.
Some phagotrophic stramenopiles.Sagenista
For the Sagenista, the phagotrophs are the Amphitremidae, amoebae protected by scales or a theca with two openings at opposite poles called pseudostomes (Figure 256). Their pseudopodia are threadlike and can anastomose. A few species harbor chlorella. It is probable that Sorodiplophrys stercorea, an amoeba capable of aggregative multicellularity by forming small sorocarps by cell aggregation, finds its phylogenetic placement in this group. The other known Sagenistas form a monophyletic line and have long been considered as atypical fungi, since they feed mainly by osmotrophy, but do not exhibit the other fungal characteristics. In addition, some species can phagocytose bacteria when they transiently differentiate from amoeboid forms. They are common saprotrophs in marine and fresh waters; some species are parasites of animals, algae or plants, the best known of which are Labyrinthula terrestris which causes damage in heavily watered golf courses and Labyrinthula zosterae which killed, a few years ago, most of the Eelgrass from the North American coast of the Pacific Ocean that served as food for ducks, leading to their starvation and great reduction in numbers. The group is characterized by the presence of a particular structure, the bothrosome (Figure 257). This bothrosome makes it possible to secrete an original “ectoplasmic” network lined with “plasma” membranes. Basal plaque appears to act in the retention of organelles in the cell body. The ectoplasmic network nevertheless seems to have the capacity to secrete various molecules. Thraustochytrida (Figure 258) are unicellular and use this network from a single bothrosome like rhizoids to degrade the organic matter on which they proliferate. Their life cycle is simple with a trophic phase that superficially resembles that of Chytridiomycota (Figure 258). Food intake is followed by the production of biflagellate zoospores typical of Stramenopila according to a monocentric eucarpic type development (Figure 258). Sexual reproduction has not been observed. In Labyrinthulida, the species are unicellular in the genus Aplanochytrium and multicellular in the genus Labyrinthula. In the latter, the ectoplasmic network is more developed and forms filaments, that serve as tracks that the ellipsoidal cells of the organism can move along. The cells are surrounded by two membranes, their plasma membrane and the inner membrane of the matrix, the sheath being delimited by the outer membrane of the matrix (Figure 259). The matrix contains polysaccharides secreted by the cells. Scales are often present between the plasma membrane and the inner matrix membrane. To achieve this structure, the membranes produced by the bothrosome must fuse. How the network is put in place, in particular how membranes merge, is still poorly understood. A partial sexual cycle has been identified in the Labyrinthula. The cells come together to form clumps. They become rounder and divide, which has been interpreted as meiosis because a synaptonemal complex is present at the first division, followed by mitosis to give eight cells. The eight cells then differentiate into zoospores possessing the two heterochontic flagella typical of Stramenopila. Plasmogamy and karyogamy have not yet been observed. Currently the Sagenista are known by a dozen species of Amphitremidae, about fifty Thraustochytrida and about twenty Labyrinthulida.
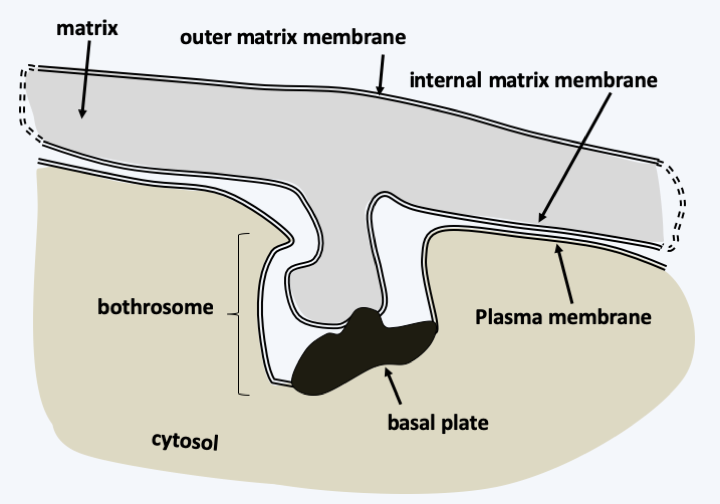
Figure 257.
Structure du bothrosome.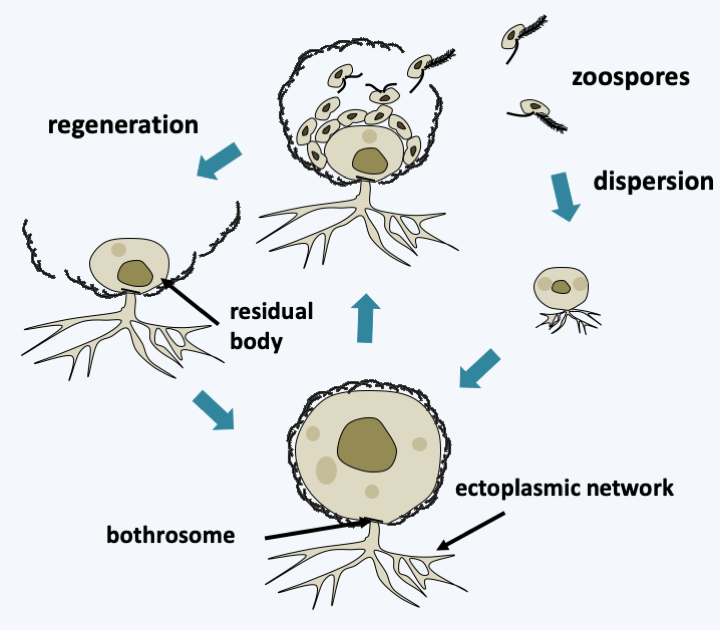
Figure 258.
Structure and life cycle of Thraustochytrida. The cycle is completely asexual. Dispersal occurs through biflagellate zoospores typical of stramenopiles. The residual body can regenerate a new individual. In some species, a motile amoeboid form differentiates before the production of zoospores.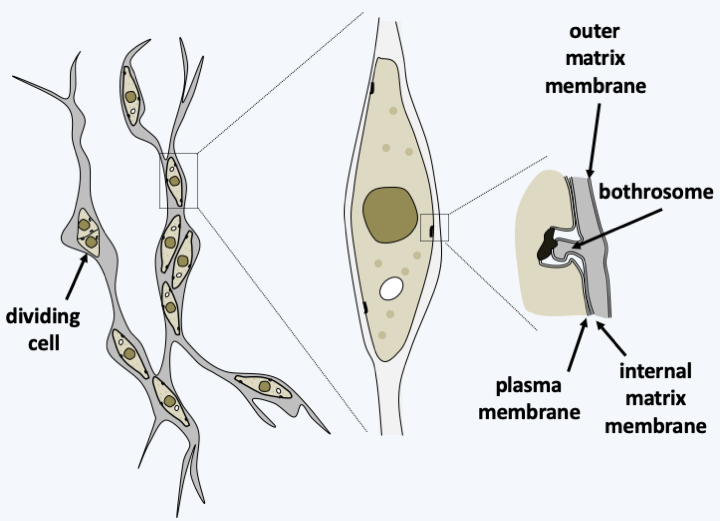
Figure 259.
Structure of the vegetative form of Labyrinthula.Opalinozoa
Phagotrophs having diverged at the base of the Opalinozoa branch are mostly flagellates with the two flagella typical of Stramenopila (Figure 256). However, structural variations may be present. For example, Incisomonas marina (Figure 256) and Solenicola setigera, the other known member of the Nannomonadea class, have only one mastigoneme-less flagellum positioned at the back of the cell, similarly as in the Opisthokonta! Silvia monomastiga, one of the smallest known phagotrophs (Figure 256), has only the anterior flagellum carrying a single row of monopartite mastigonemes. This flagellum has only doublets of microtubules left in the basal body. In some species, the cell in its anterior part can be deformed by amoeboid movements. Some are protected by thecae. They divide by binary fission, sexual reproduction not being known. They are very abundant organisms. Cafeteria roenbergensis (Figure 256) is one of the 20 most common plastid-free flagellates in marine waters. The presence of variable morphologies makes it possible to define several lines of these phagotrophs, whose divergence is probably very old, which is confirmed by the very different sequences of their genes. It is estimated that their genetic diversity is as great as that of metazoans! Likewise, the described morphospecies probably hide several cryptic species. Molecular phylogenies presently divide these Opalinozoa flagellates into three monophyletic lineages, the class of Nannomonadea which contains two described species, the Placididea which contains three, and Bikosea that contains a few dozen.
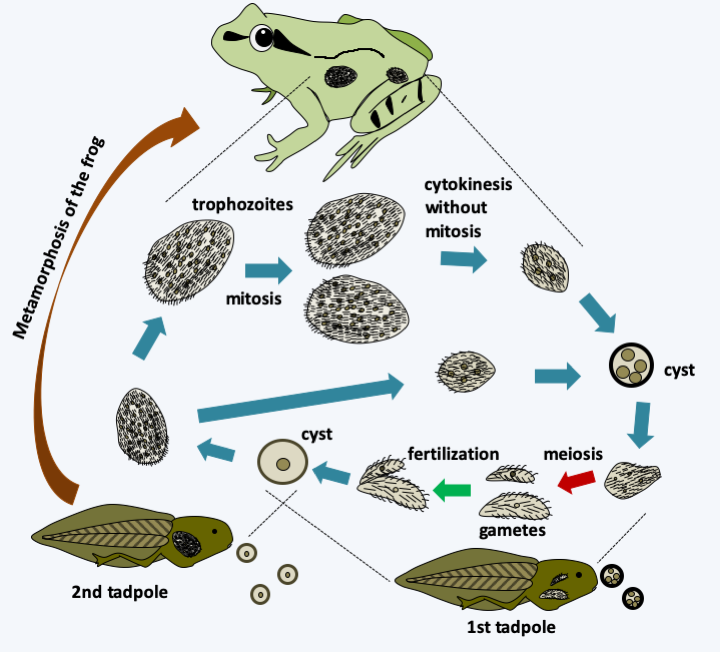
Figure 260.
Opalina ranarum cycle. Cysts secreted by adult frogs are ingested by a first tadpole, in which they wake up and migrate into the cesspool. Meiosis and the formation of male and female gametes occur. They are followed by fertilization which produces cysts which are excreted. Ingested by a second tadpole, they hatch to give rise to small trophozoites (~ 500 μm), which can give rise directly to cysts. After metamorphosis of the frog, trophozoites reach larger sizes (~ 3 mm) and can contain up to 2000 nuclei. During the frogs' breeding season, sex hormones cause cell divisions without growth or mitosis. The cells which have between two and twelve nuclei form the cysts which are secreted.The last class of Opalinozoa, that of Opalinata, contains organisms living in commensal or parasitic symbioses with animals. There are about 400 species divided into two subclasses. Opalinea are commensals of the terminal part of the intestine of cold-blooded vertebrates, especially frogs. They are large cells that can measure up to 3 mm, plurinucleated and covered with a regular and particular ciliature. They are anaerobic and feed by pinocytosis of the fluids present in their biotope. The Opalina ranarum life cycle alternates between the adult frog and the tadpole (Figure 260). Blastocystea are commensals or parasites, like Proteromonas lacertae and Blastocystis. Proteromonas lacertae is an anaerobic biflagellate that lives in the terminal intestine of lizards and has a unique structure (Figure 261). Blastocystis are parasites of the intestines of animals, including humans. They lack a flagellum and other characteristic structures. Only sequence data has made it possible to classify them among the Opalinozoa. Like Proteromonas lacertae, Blastocystis species are anaerobic and live in the intestines of vertebrates and invertebrates. Their mitochondria are modified and have characteristics specific to normal mitochondria and others specific to hydrogenosomes. They indeed have a partial citric acid cycle and an iron hydrogenase. Under a relatively uniform morphology, hides a great genetic diversity. Their life cycle takes them through several forms (Figure 262). The human parasite, Blastocystis hominis, is not very dangerous because most carriers are asymptomatic. The others have bloating, mild diarrhea, abdominal pain and possibly cramps. These organisms can now be treated with antiprotozoa.
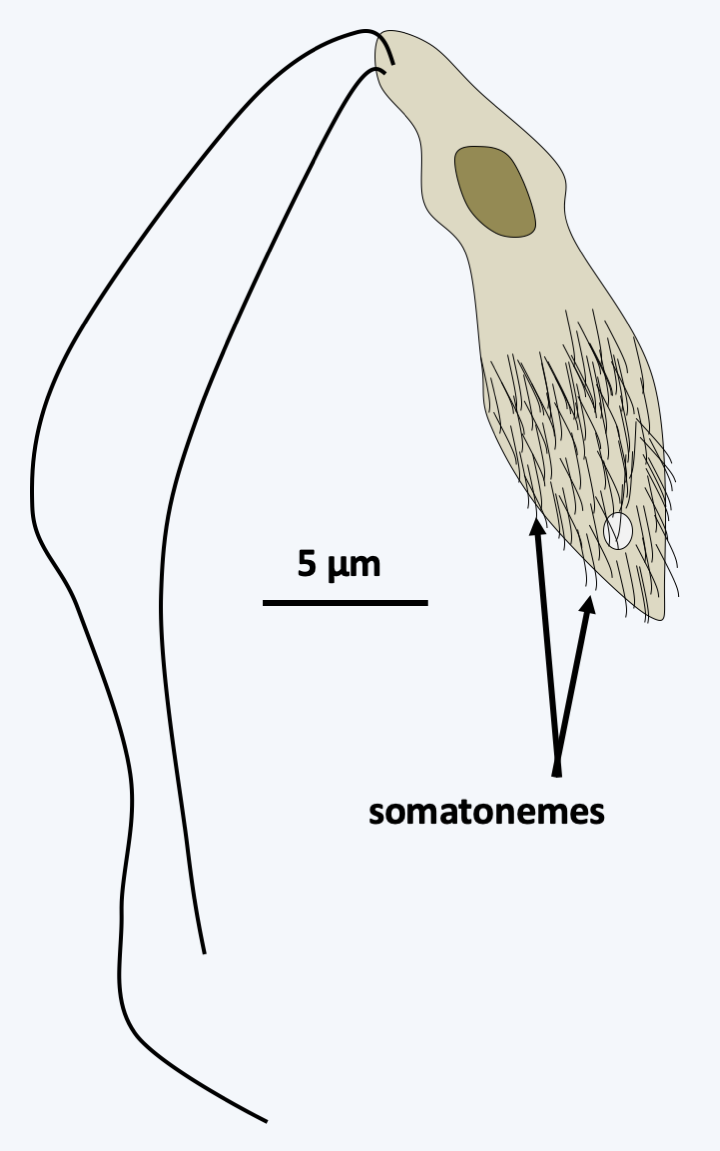
Figure 261.
Proteromonas lacertae. This flagellate has somatonemes on the posterior two-thirds, whose structure resembles that of mastigonemes. They could be counterparts. The flagella appear to be devoid of mastigonemes.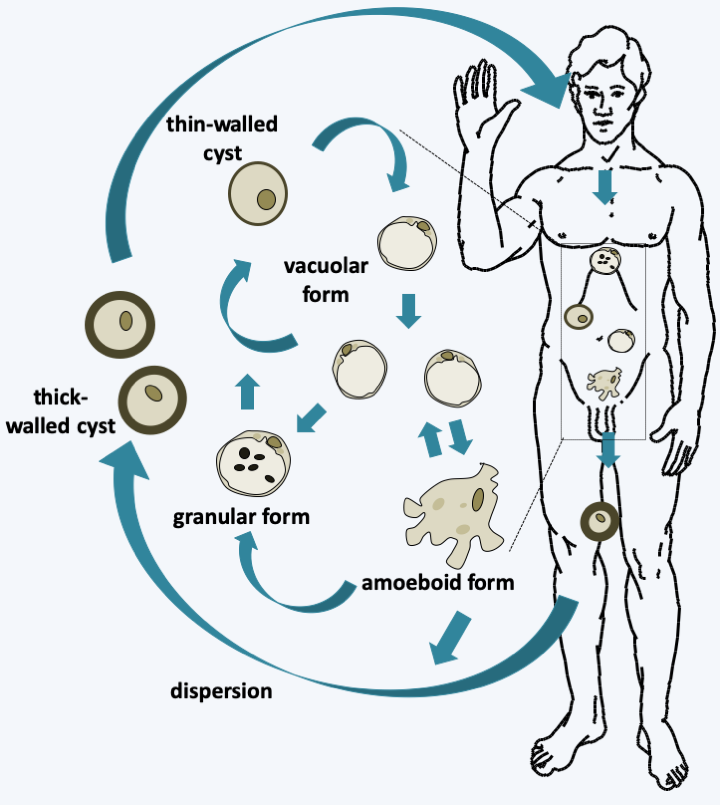
Figure 262.
Blastocystis hominis cycle. Ingestion of cysts via contaminated food causes their wall to rupture and the vacuolar form to awaken, which presents a large central vacuole. This vacuolar form appears to be the main trophic form. It can be differentiated into amoeboid or granular forms, the roles of which are obscure. Cysts are then differentiated; thin-walled ones appear to be involved in autoinfection and thick-walled ones in dispersion and persistence in the environment.Gyrista
The Gyrista lineage encompasses the vast majority of Stramenopila species (Figure 254). The earliest diverging group of the lineage consists of free phagotrophs possessing the two flagella typical of Stramenopila. They are classified in the class of Developpayellida, the best characterized species of which is Developayella elegans (Figure 256). The other lineages are generally not exclusive phagotrophs, as some of these organisms have secondary endosymbiosis with a Rhodophyta, rendering them photosynthetic, and others are osmotrophic fungal-like organisms. The modalities of the endosymbiosis are still debated. Some believe that a single endosymbiosis gave rise to the Cryptophyta, Haptophyta and Ochrophyta, which are the photosynthetic Stramenopila. The lineages devoid of plastids would therefore have lost them. Their main argument is that plastids have the same structure in all three classes: they are surrounded by four membranes, the last of which is in fact that of the reticulum / outer membrane of the nucleus and all of them originate from a Rhodophyta. Opponents of this theory point out that horizontal transfer of the rpl36 plastid gene, which characterizes Cryptophyta and Haptophyta, is absent in Ochrophyta. It would therefore be necessary to imagine that the transfer took place in a plastid surrounded by four membranes, which is quite unlikely. Above all, they point out that to explain the current phylogeny, it is necessary to imagine multiple independent losses in many groups, which is also very unlikely. The problem is compounded, however, when the genomes of Oomycota, close relatives of Ochrophyta that have adopted a fungal lifestyle and are devoid of plastids, are analyzed. Indeed, genes whose function is typically associated with that of the plastids are present in relatively large numbers in their genome. These organisms could therefore have effectively had a plastid and lost it. The alternative hypothesis is that the genes in question could have been acquired by horizontal transfer from prey by their phagotrophic ancestors. Likewise, molecular phylogenies indicate that Actinophryida, which are phagotrophic heliozoans, have Ochrophyta algae from the Raphidophyceae class as ancestors! Anyway, molecular phylogenies show (Figure 254) that the Ochrophyta algae form a monophyletic set that diverges from a lineage, the Pirsoniida, whose current members have adopted a parasitic lifestyle while being capable of phagotrophy. This lineage emerges from a collection of other organisms with fungal life styles known as Pseudomycota. These “false fungi” evolved in parallel with the Eumycota and acquired the same properties during their evolution. For a long time they were classified with the Eumycota and it was thanks to molecular phylogenies that it was possible to separate them, although many mycologists had long considered that some of their characteristics were very different from other fungi and that as such they deserved to to be separated from Eumycota. Molecular phylogenies currently define two monophyletic lines of Pseudomycota (Figure 254): Oomycota and Hyphochytridiomycota. The differentiation between these groups is supported by them having different characteristics.
Oomycota
The 600 species of Oomycota had an evolution very similar to that of Eumycota. As in Eumycota, the more “primitive” species have vegetative development similar to that of Chytridiomycota and the more “evolved” species appear as a multinucleated mycelium. However, this mycelium is aseptate and the diameter of the hyphae is usually larger than that of Eumycota hyphae. These organisms are common in fresh or salt water and soils, where many species live as saprotrophs. There are also many parasitic species of animals or plants. As with the Eumycota, different lifestyles have been adopted convergently by the different lineages in Oomycota. Unlike Eumycota, their cell wall contains cellulose. There is no yeast form, nor a complex multicellular sporophore, like a mushroom. Their life cycle is of the diplobiontic type. Lysine synthesis occurs via the diaminopimelic acid pathway and as with other Stramenopila, their reserves are made of laminarins. Molecular phylogenies confirm several orders defined on morphological and physiological criteria (Figure 263).
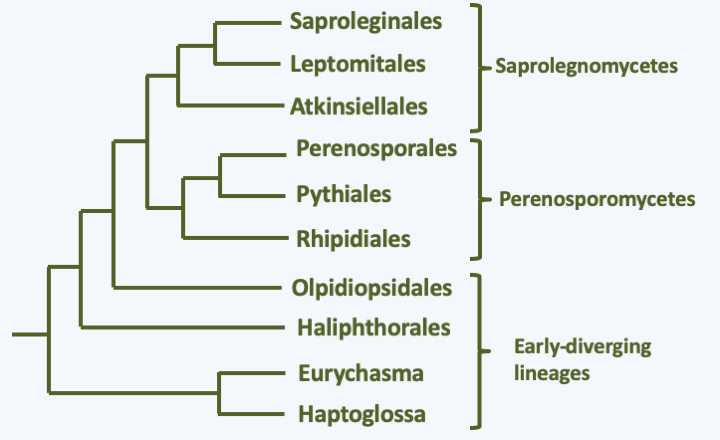
Figure 263.
Phylogeny of Oomycota.At the base of the tree, a monophyletic line unites the Haptoglossa and the species Eurychasma dickinsoni (Figure 263). While Eurychasma dickinsoni clearly possesses typical fungal biology, Haptoglossa are sufficiently dissimilar to merit separate treatment. Species of this genus are single-celled parasites of nematodes and rotifers that have developed a very sophisticated 15-micron cell to get inside their host: the gun cell (Figure 264). This mechanism allows the parasite to forcefully penetrate the nematode in the same way that Microsporidia penetrate their host or Cnidaria cnidoblasts inject their poison. Once in the nematode, Haptoglossa form one or more multinucleated thalli surrounded by walls and which eventually differentiate into a sporangium. In some species, the spores are motile and encyst, while in others they are non-motile. When differentiated, the zoospores are biflagellate but do not appear to exhibit the typical mastigonemes of the group; it seems, however, that some species have some residual mastigonemes at the base of one of the flagella. The only species in the genus Eurychasma, Eurychasma dickinsoni, infects a large number of species of Phaeophyta algae. It has a holocarpic cycle that strongly resembles that of early diverging fungi such as Chytridiomycota. Compare the life cycle in Figure 119 with that of Eurychasma dickinsoni shown in Figure 265…
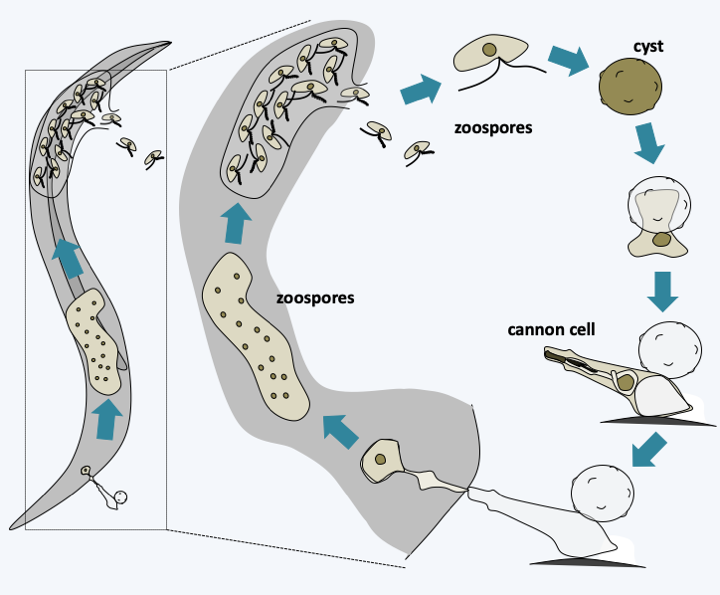
Figure 264.
Haptoglossa mirabilis life cycle.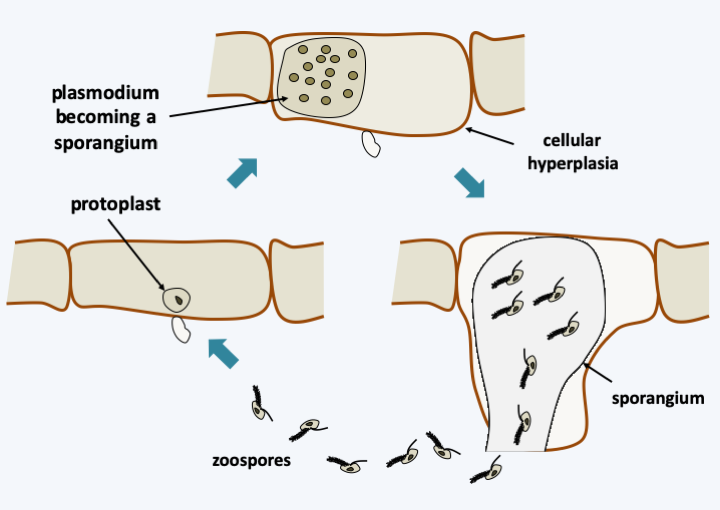
Figure 265.
Eurychasma dickinsoni cycle. The known cycle is asexual. Entry into a Phaeophyta algae cell leads to the development of a plasmodium which causes hyperplasia of the host cell. This then differentiates into a sporangium which releases heterochontic biflagellate zoospores typical of Stramenopila.A holocarpic development is also adopted by several “primitive” species belonging to the orders Haliphthorales and Opsidiopsidales and which diverged just after the Haptoglossa/Eucharysma (Figure 263). These marine Oomycota parasitize crustaceans or Rhodophyta respectively. Their development is often poorly understood, for example, sexual reproduction for these organisms has not been described. The other species fall into two broad classes, Saprolegniomycetes and Perenosporomycetes. The former are generally saprotrophs or parasites of freshwater animals and the latter are generally pathogens of plants, crustaceans or nematodes, and therefore very present in terrestrial ecosystems. There are of course many exceptions and some Saprolegniomycetes, such as Atkinsiela dubia, parasitize marine crustaceans and Perenosporomycetes such as Sapromyces elongatus is a soil saprotroph. It is not clear, for example, if the sea is the original habitat of the two great lineages or if marine species have re-invaded this environment. These organisms differentiate mycelia resembling those of zygomycetous fungi without septa or anastomoses. Their life cycle is of the diplobiontic type with a phase of asexual reproduction, with dispersion ensured by biflagellate zoospores characteristic of Stramenopila, and a phase of sexual reproduction called oogamy, because of the involvement of a female gamete resembling an egg. The sporophores they make are simple and never multicellular. The type species of the group is Saprolegnia parasitica, a parasite of fish, amphibians and crustaceans, important in aquaculture. Its life cycle is well studied (Figure 266). The peculiarities of this diplobiontic cycle and the difficulty in cultivating and inactivating genes by deletion have meant that the acquisition of knowledge about Oomycota has not progressed as much as that about Eumycota. In addition, the sequencing of genomes of several species shows a coding capacity greater than that of Eumycota. The typical Oomycota genome appears to contain 25,000 genes spread over a genome measuring 75 Mb, double the coding capacity of typical Eumycota. Members of the Pythiaceae family of the order Perenosporales exhibit a unique characteristic in eukaryotes in that they are incapable of making sterols, an in fact do not need them in the medium for vegetative growth. However, sterols are required for sexual reproduction and maturation of sporophores.
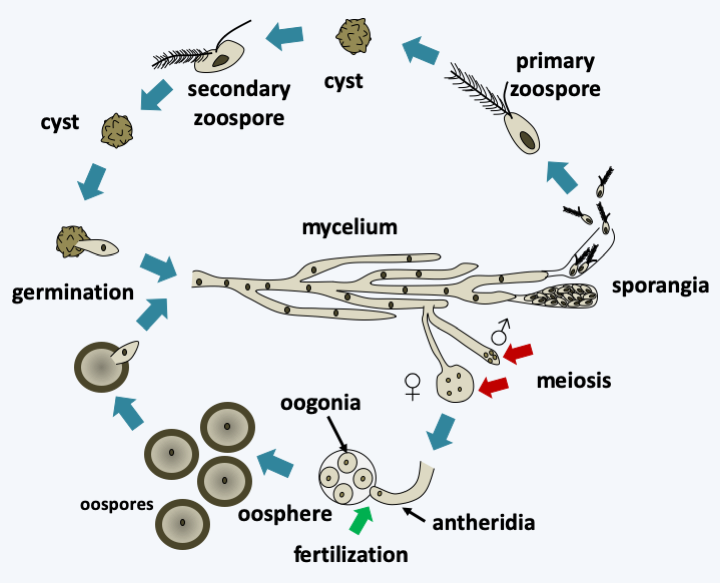
Figure 266.
Saprolegnia parasitica cycle. This species exhibits a cycle typical of the Saprolegnomycetes. There is a phase of asexual reproduction where the mycelium differentiates at the end of the hyphae from sporangia containing zoospores which have the flagellature typical of stramenopiles. The production of zoospores depends on varying environmental conditions depending on the species. These zoospores can become encysted and give rise either directly to a mycelium or to a secondary zoospore with a slightly different structure and which after encystment gives rise to a mycelium. In some Oomycota only one type of zoospore is observed. As in most Oomycota, the hermaphroditic mycelium can differentiate between male and female reproductive structures. Meiosis takes place in these specialized structures. The male structure or antheridium is attracted to hormones emitted by the female structure or oosphere containing oogonia. It emits a germ tube which ensures fertilization inside the female structure. The formed zygote develops into a large egg-like oospore. Generally, the species are homothallic, exceptionally they are heterothallic.Besides Saprolegnia parasitica, the Oomycota tree has a few branches harboring major pathogens of considerable economic importance. Phytophtora infestans attacks potatoes and tomatoes and was the cause of a famine in Ireland in the summer of 1846 that killed a million people and pushed 1.5 million others to emigrate. This is the last great famine in Europe. Phytophtora infestans grows in stems and leaves but also attacks tubers. Under ad hoc conditions such as the temperate European climate in “rotten” summers, it grows rapidly. It seems that a week was enough for the complete destruction of potato production during the Irish famine. This species is still causing havoc! Plasmopara viticola is a parasite of the vine that causes “mildew”. Accidentally introduced into Europe at the end of the 1870s via American vine strains that were resistant to an aphid that was ravaging vineyards in France, it destroyed almost all French wine production at the time… The discovery that the mixture of limestone and copper sulphate is active on this Oomycota made it possible to develop the first chemical product controlling a plant parasite: Bordeaux mixture.
Hyphochytridiomycota
There are about twenty species belonging to the line of Hyphochytridiomycota. They are characterized by the loss of the posterior flagellum of the Stramenopila, and only the anterior flagellum, carrying mastigonemes, remains. However, the basal body of the second flagellum is still present. These cosmopolitan organisms live as saprotrophs in soils or waters and in mutualistic or parasitic associations. For example, Hyphochytridium catenoides is common in soils but is also found associated with Oomycota spores or conifer pollen grains which it parasitizes. Rhizidiomyces apophysatus parasitizes Oomycota oogonia. The asexual cycle of these organisms resembles that of the Chytridiomycota or Oomycota (Figure 267). The zoospore attaches and differentiates into cysts. It produces rhizoids that penetrate the host. Inside, a thallus can form and differentiate various structures including sporangia, which develop depending on the species in a holocarpic, polycarpic monocentric and polycarpic polycentric manner. So far sexual reproduction has not been described in the group.
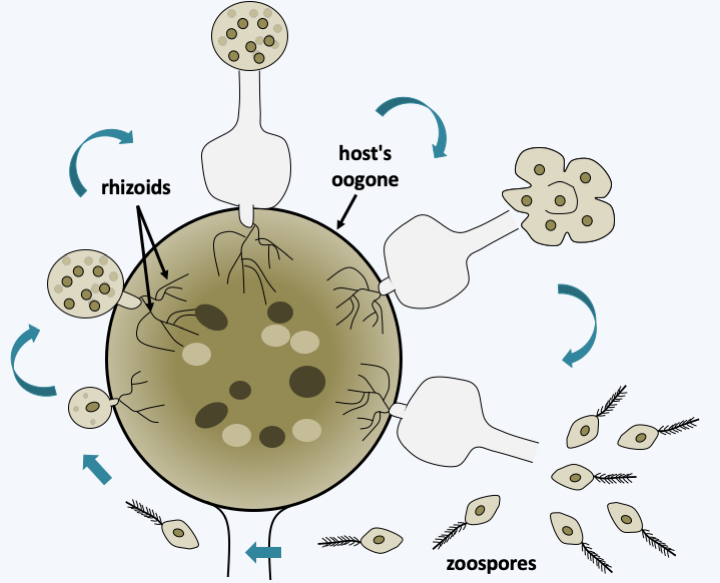
Figure 267.
Rhizidiomyces apophysatus cycle. In this species, the precursor 'protoplasm' of the zoospores is expelled outside the sporangium before the spores are fully mature.Pirsoniida
The few known species of Pirsoniida are diatom parasites or predators. Indeed, after having penetrated the frustule of their host, they consume the cell body by phagocytosis of small pieces of the “protoplasm”, inevitably leading to the death of their host / prey (Figure 268). They disperse via zoospores typical of Stramenopila. Molecular phylogenies place the Pirsoniida close to Hyphochytridiomycota and, like them, they are devoid of plastids. Nevertheless, some phylogenies place them, as shown inFigure 254, as a sister group of photosynthetic Ochrophyta. The resolution of their phylogenetic position, as well as knowledge of the presence of a plastid relic in these organisms would allow us to conclude on the origin of the plastid in Ochrophyta and therefore probably also in Haptophyta and Cryptophyta. Indeed, if their position as a sister group of Ochrophyta and the absence of plastid - or of a genomic or cytological trace of their presence in the past - are confirmed, the unique origin of endosymbiosis in Ochrophyta, Haptophyta and Cryptophyta will become difficult to sustain!
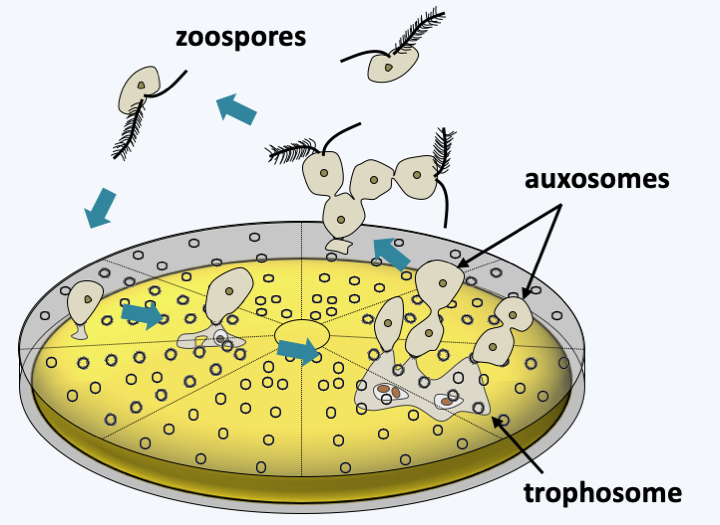
Figure 268.
Cycle of a Pirsoniida. After penetration of the frustule, the trophosome allows the Pirsoniida to phagocytize the cellular content of the diatom. The rest of the cell body forms auxosomes which eventually differentiate into zoospores ensuring dispersal.The last lineage of Stramenopila currently known from data other than those of metagenomics is that of Ochrophyta. The diversity of the organisms that compose it, as well as their importance in ecosystems, require treatment in a separate chapter.
Back to chapter index