Excavata
Back to main indexJump to section:
Introduction
It is likely that no other lineage of eukaryotes has posed such a problem for biologists as that of the “Excavata”. The excavates comprise three groups: Metamonada, Malawinmonada, and Discoba. The three groups were originally considered to be monophyletic based on the presence of a ventral groove where food is phagocytosed. However, molecular phylogenies show that the exacavates are not monophyletic, and the the relationships of the three sub-clades to other eukaryotes remain uncertain. The main reason for the uncertainties about the phylogeny of the “Excavata” is probably due to their very great genetic diversity. Figure 198 gives the current phylogeny.
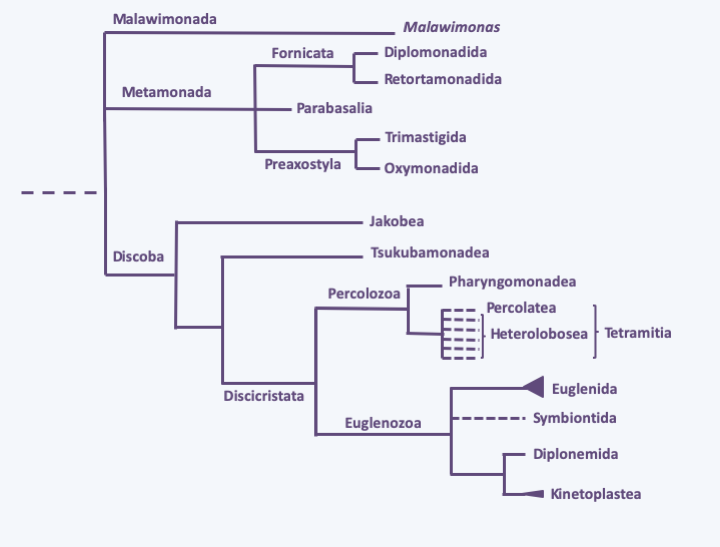
Figure 198.
Phylogeny of Excavata.Metamonada
The Metamonada phylum includes a set of anaerobic protists, some of which live free in sediments, or water laden with organic matter, while others live in mutualistic or parasitic associations with animals. Due to their lifestyles these organisms have reduced mitochondria that have become either hydrogenosomes or mitosomes. Three Metamonada classes are currently recognized, based primarily on molecular phylogenies and arrangement of flagella.
Fornicata
The Fornicata class contains a few hundred species. Its classification is very fluctuating and it is generally split into two subclasses, Diplomonadida and Retortamonadida. They are heterotrophic, single-celled flagellated protists with a nucleus typically associated with a specific microtubule network, all referred to as a “karyomastigont” (Figure 199). A few live free in water that is rich in organic matter and poor in oxygen, but the majority live as commensals or parasites of the intestines of animals. For example, Spironucleus salmonicida (Figure 199) is a parasite of salmon. All biological data indicate that these organisms are very simple, because their internal structure shows an absence of mitochondria, and in some species at least an absence of Golgi apparatus, peroxisomes etc. The human parasitic species Giardia intestinalis (= Giardia lamblia = Giardia duodenalis) is the most studied Fornicata (Figure 200). This species is very common in underdeveloped countries, in particular in orphanages, but also in certain large cities of the United States, Australia or Moscow. The addition of chlorine does not kill it. Very infectious, it is transmitted through the ingestion of food soiled by the faeces of infected people. Incubation lasts from one to four weeks. It causes non-fatal diarrhea which is often self-healing: it is a major cause of diarrhea worldwide. Besides simple metabolism, having only one mitosome, the genome sequence of Giardia intestinalis has shown reduced coding capacity. The genome measures 12 Mb spread over five chromosomes and encodes around 6,500 genes. Most of the molecular complexes involved in the replication and expression of genetic information are simple because they have a small number of subunits. The genome codes for the RNA splicing machinery, but so far only four introns have been identified! Giardia intestinalis also has a particular cytoskeleton. There are indeed microtubules and the flagella are typical of eukaryotes, but there does not appear to be any dynein. Likewise, the genome has a gene encoding actin but does not have genes encoding proteins associated with microfilaments, including myosin. The genome of Spironucleus salmonicida shows greater complexity in terms of both information expression and metabolism; this species has a hydrogenosome. In summary, all the data indicate that Giardia intestinalis and the other Fornicata are simple organisms deriving from a reductive evolution like what has happened in many other parasites.
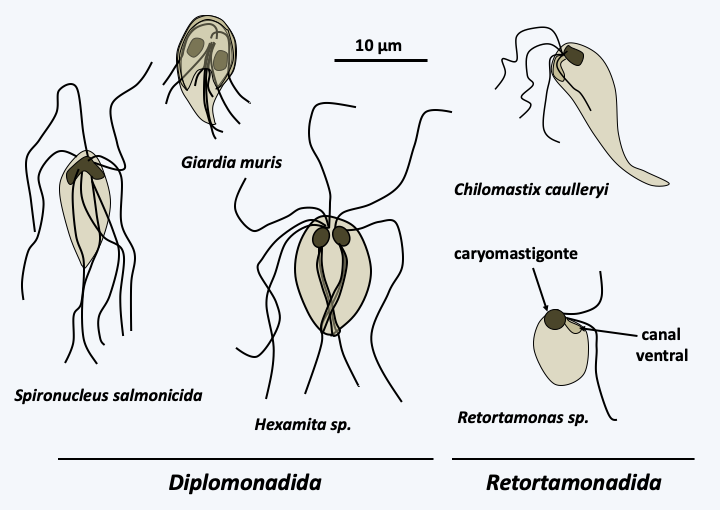
Figure 199.
Some Fornicata.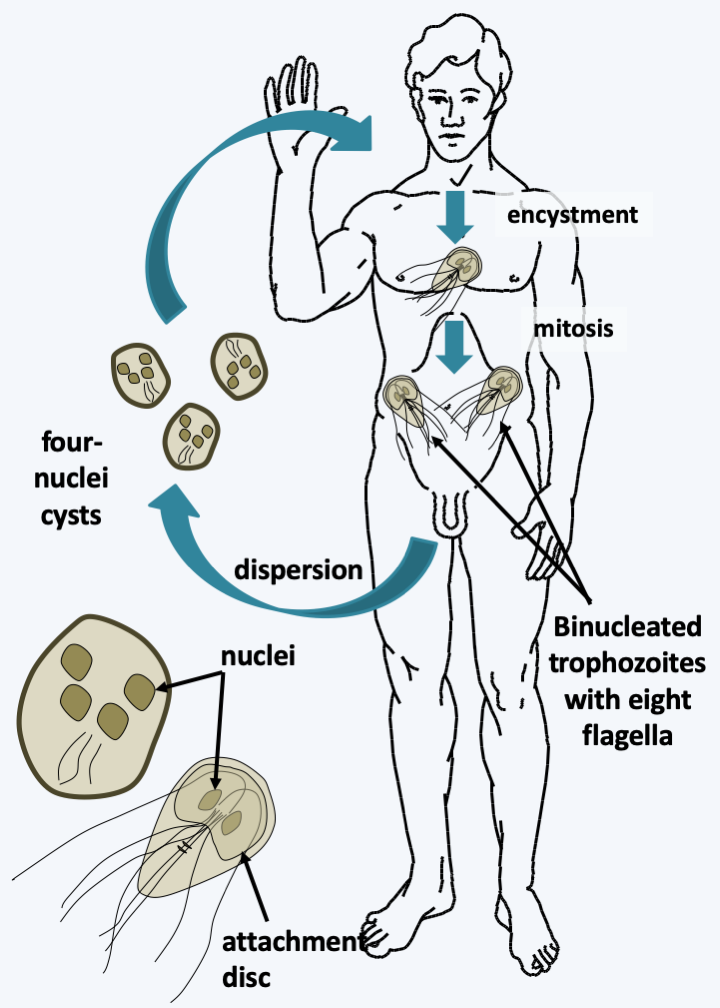
Figure 200.
Giardia intestinalis cycle. The cycle is simple with no known true sexuality. The division takes place by binary fission, which requires the attachment of the parasite to the mucosa via an adhesive disc; otherwise it swims rapidly in the intestinal lumen. The free forms are differentiated into cysts resistant to desiccation and allowing dissemination outside the body. Cysts enter the host and develop into trophozoites. The trophozoite measures 10-15 μm and has two identical nuclei, transcriptionally active and linked to four pairs of flagella. No real sex cycle has been observed, but the presence of genes typically involved in meiosis in the genome suggests that these organisms were sexed in the past. These genes would now be involved in a karyogamy process allowing the exchange of genetic material between the two nuclei. These exchanges would explain why the diploid genome of Giardia intestinalis has few polymorphisms between the copies of the genome present in each of the nuclei.Parabasalia
The Parabasalia class contains about 400 species. These organisms have varied morphologies ranging from flagellated to amoeboid forms (Figure 201), which eventually change depending on external conditions or stage of development. They are initially characterized by an arrangement of the karyomastigont that is different from that of the Fornicata, with the four basal bodies and supporting fibers connected to the Golgi apparatus, defining a structure called the “parabasal body” which gave the class its name. On this model there are many variations in the number of flagella, ranging from zero to several dozen. The parabasal body is associated with a particular microtubular structure, the axostyle (Figure 201). In addition, the number of flagella may change depending on their stage of development, so that different forms of the same species have been classified into separate genera. In most species, there are no forms of resistance such as cysts. Parabasalia therefore live in association with animals either as commensals such as Trichomonas tenax and Trichomonas hominis which live in the mouth in humans, or as parasites such as Trichomonas gallinae or Histomonas meleagridis which attack birds, or even as mutualistic like the species found in the digestive system of termites. The latter themselves live in mutualistic symbiosis with bacteria, which affect the hydrolysis of cellulose, ultimately allowing termites to feed on wood. Most Parabasalia have hydrogenosomes, the functioning of which is described in Figure 31.
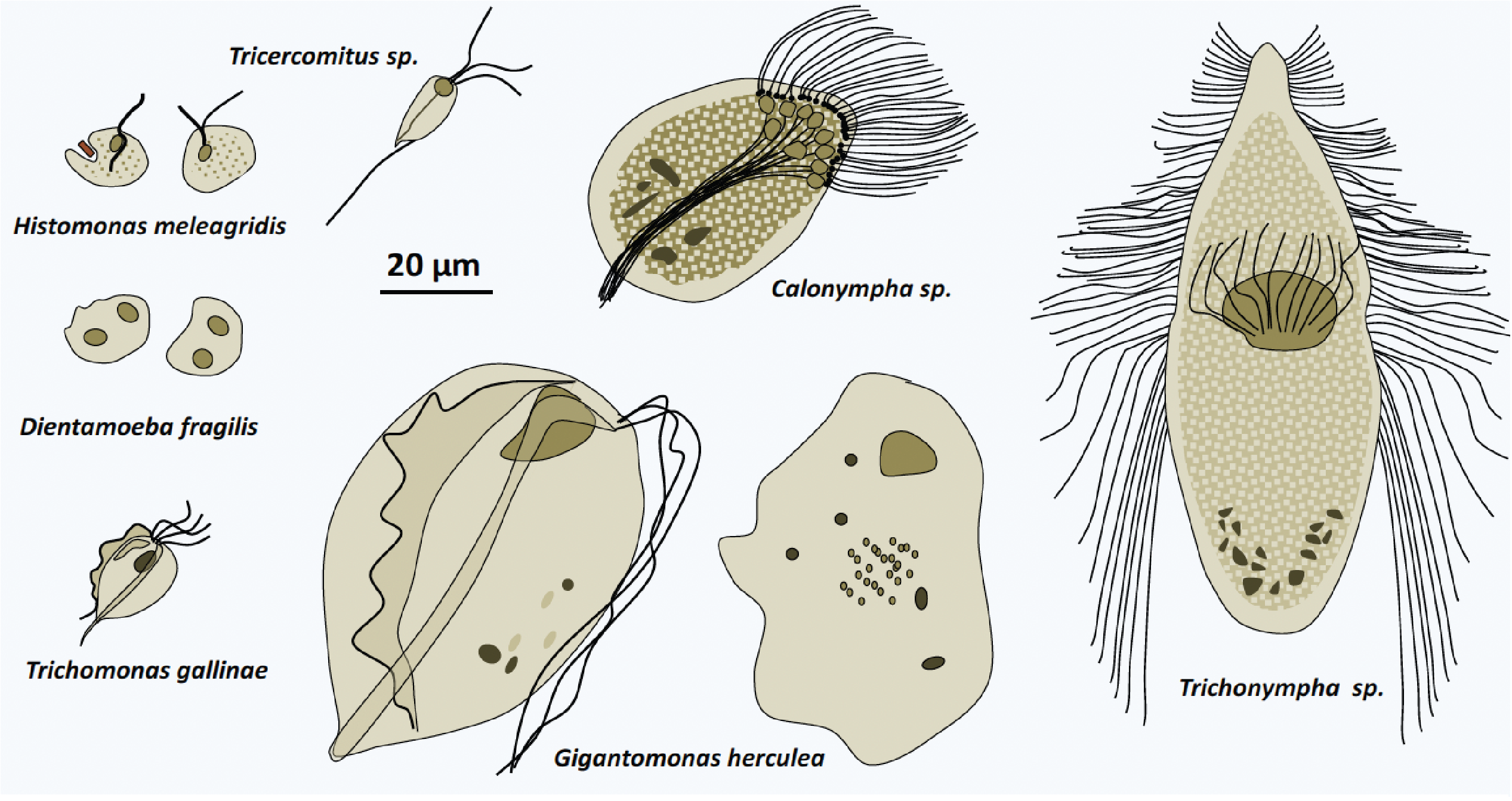
Figure 201.
Diversity of Parabasalia.The most studied Parabasalia species is Trichomonas vaginalis, which is responsible for the sexually transmitted disease trichomoniasis (Figure 202); these Parabasalia are part of the species that do not form dispersal and resistance propagules, they need an environment that is always humid to be transmitted. The symptoms are especially present in women with vaginitis and urethritis. Men are often a healthy carrier, but the parasite can cause urethritis. Trichomoniasis is the most common non-viral sexually transmitted disease, affecting about a quarter of women in their lifetime. It is present all over the world. The Trichomonas vaginalis genome, which measures 160 Mb, contains 65% repeated sequences and, very surprisingly, around 60,000 protein coding genes, twice as many as in humans! A rare feature of this genome is that it does not appear to contain a gene encoding myosin, like that of Giardia intestinalis. About 150 genes come from horizontal transfers from bacteria; an indication that the ancestors of this species were phagotrophs, having since moved on to feed only by osmotrophy. Like the Fornicata, the Parabasalia therefore derive from free phagotrophs by regressive evolution.
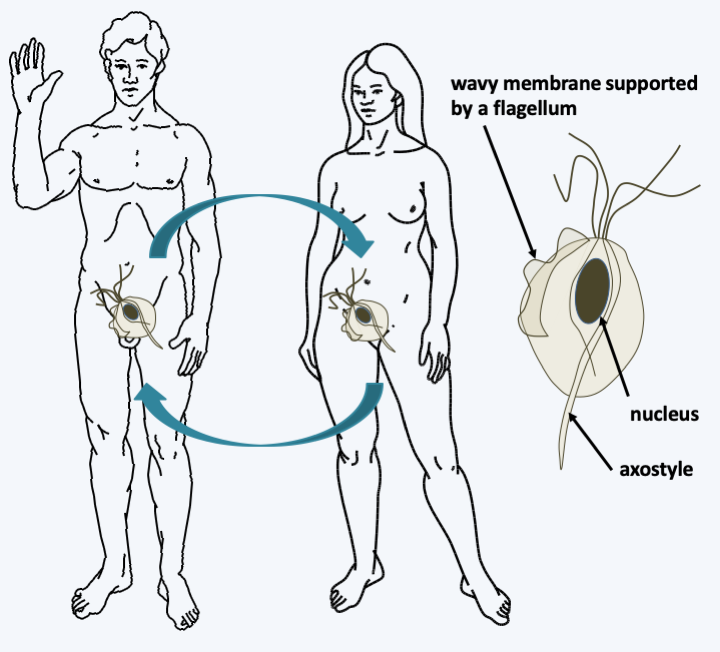
Figure 202.
Structure and cycle of Trichomonas vaginalis. The trophozoites are between 10 and 23 μm. Besides the hydrogenosomes, they have between three and five flagella, as well as a Golgi apparatus associated with the fibers forming the parabasal body, the characteristic structure of the group. The axostyle is a microtubular structure associated with basal bodies involved in cell motility and/or structuring. Trichomonas vaginalis has a simple cycle devoid of sexuality or resistance formation; reproduction occurs only by binary fission. Trichomonas vaginalis needs a pH of 4 to develop. Note that during mitosis in this species as in other Parabasalia, the mitotic spindle lies outside the nucleus and attaches to the centromeres via the nuclear membrane.Preaxostyla
The third and currently last class constituting the Metamonada, that of the Preaxostyla, contains a few dozen flagellated anaerobic protists (Figure 203). Members of the subclass Trimastigida are free-living, while members of the Oxymonadida subclass form obligatory mutualistic symbioses in the intestines of animals, mainly termites and cockroaches. Like the Parabasalia, Preaxostyla participate in the degradation of wood. They are characterized by their four flagella, the ventral feeding groove typical of Excavata, hydrogenosomes, an asymmetric cytoskeleton including a particular microtubular structure called preaxostyle. This structure resembles the axostyle of the Parabasalia and like it is used for swimming and cellular support. Preaxostyla have hydrogenosomes but their Golgi apparatus is reduced. Little is known about these organisms, as they are very difficult to cultivate.
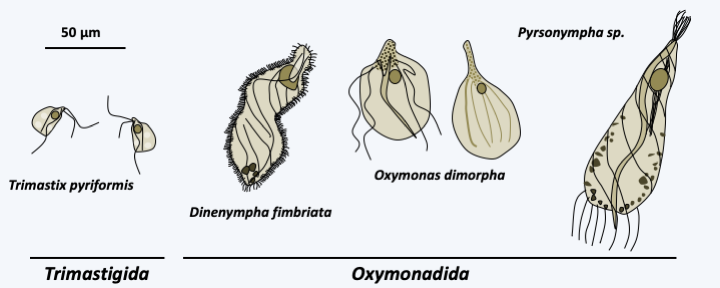
Figure 203.
Some Preaxostyla.Discoba
The Discoba phylogeny has been defined primarily on the basis of molecular phylogenies which indicate it is monophyletic. The first two classes of Jakobea and Tsukubamonadea only include a few species but whose biology is interesting.
Jakobea
The Jakobea class contains a dozen species of flagellates, all of which are classified in the order of Jakobida. These are bacterivorous biflagellate cells that live in fresh or marine waters (Figure 204). They can swim freely or be attached to a substrate. Some are protected by a lorica, which is a basket-shaped test. They have a well-developed ventral feeding groove and a karyomastigont associated with a Golgi apparatus with a single dictyosome (cup-shaped array of flattened membranous vesicles). Jakobida reproduce by binary fission; a sexual cycle has not been observed. Their most remarkable property is their mitochondrial genome which contains a very large number of genes and clearly retains the properties of a prokaryotic genome. The structure of the mitochondrial genome therefore suggests that these flagellates have changed little during evolution and therefore closely resemble the ancestors of the eukaryotic cell. Their diet and their free lifestyle would therefore confirm the theory of the phagotrophic origin of the eukaryotic cell. For a long time, the Malawimonadida and the Diphyllatea which have similar biology were classified with the Jakobea. Molecular phylogenies however place them at the base of the radiation of Amorphea. This position seems to confirm the hypothesis that this type of flagellated eukaryotes, with a karyomastigont associated with the Golgi apparatus and a ventral groove participating in the phagocytosis of bacteria, is the most “primitive”.
Tsukubamonadea
The Tsukubamonadea class currently has only one species (Figure 204) classified in the order of Tsukubamonadida and whose biology and morphology are comparable to that of the Jakobea. Molecular phylogenies clearly differentiate it from Jakobea, which is confirmed by the structure of its mitochondrial genome, which encodes twice as many genes as that of Reclinomonas americana.
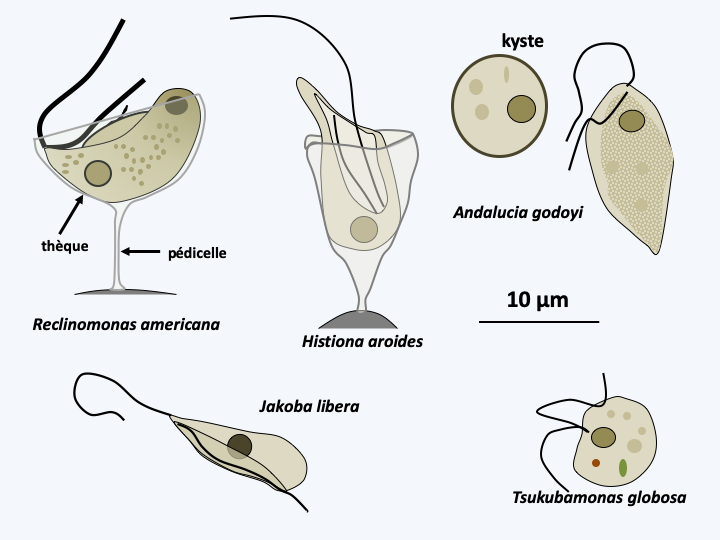
Figure 204.
Some Jakobea and Tsukubamonadea.Discicristata
The super-branch of the Discicristata groups together two branches, that of the Percolozoa, also called “Heterolobosea sensus lato” and that of the Euglenzoa. Discicristata includes around 1,400 species, all of which exhibit mitochondria with discoid ridges. With 140 described species (Figure 205), the branching of Percolozoa is poorly understood. They are mostly small, colorless, uninucleated cells that are adapted to life in many environments, including extreme halophilic, acidophilic or thermophilic ones. One lineage has adapted to life under anaerobiosis and has hydrogenosomes that appear to function similarly to Parabasalia. Most species can be differentiated either in an amoeboid form whose pseudopodia are “eruptive”, that is, they form suddenly, or in flagellate form, or in cysts. The transition from the amoeboid form to the flagellated form occurs at the time the organism meets water, in order to be able to swim. The flagellate form does not divide and usually does not feed. It must therefore regain an amoeba form to take in food. The number of flagella varies from two to several hundred in species of the genus Stephanopogon (Figure 205). Cysts are very resistant, their survival can last several decades. The two best known species are Naegleria gruberi and Naegleria fowleri. The first serves as a laboratory model to study the transition between the amoeba form and the flagellate form (Figure 206). The second is responsible for amoebic meningoencephalitis, a rare but fatal disease. It usually lives in hot stagnant water at 35°C and is caught by contact with the nasal mucous membranes. It is rare in Europe except in hot water at the exit of nuclear power plants or in poorly maintained swimming pools… It enters the cerebral cortex, which it quickly destroys, causing death in less than a week. Currently, there is no cure. This infectious process is a dead end because the amoeba has no mechanism to spread once the patient dies. Infections are therefore caught randomly and do not have an epidemic aspect. These amoebae are not a public health problem, but are closely monitored by EDF (Electricity of France)… One lineage, that of Acrasida, evolved an aggregative multicellularity with differentiation of simple sporophores, but having a characteristic morphology (Figure 207).
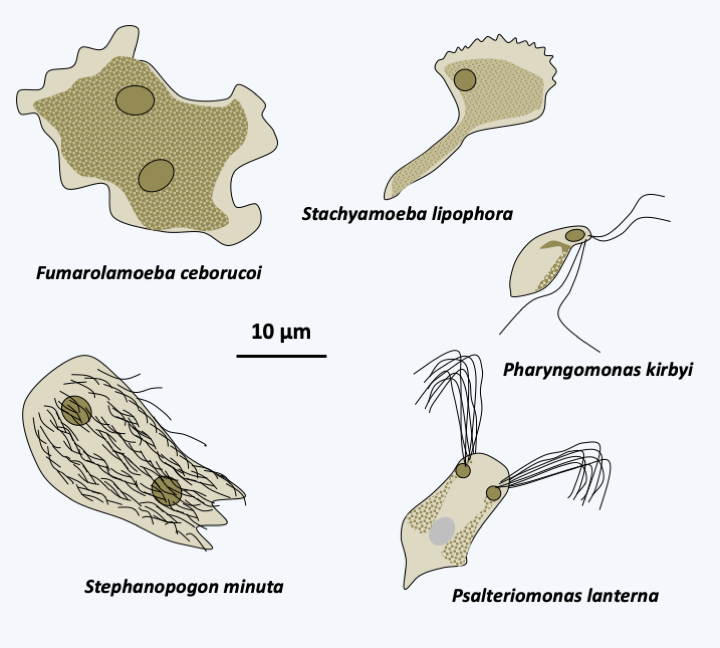
Figure 205.
Diversity of Percolozoa.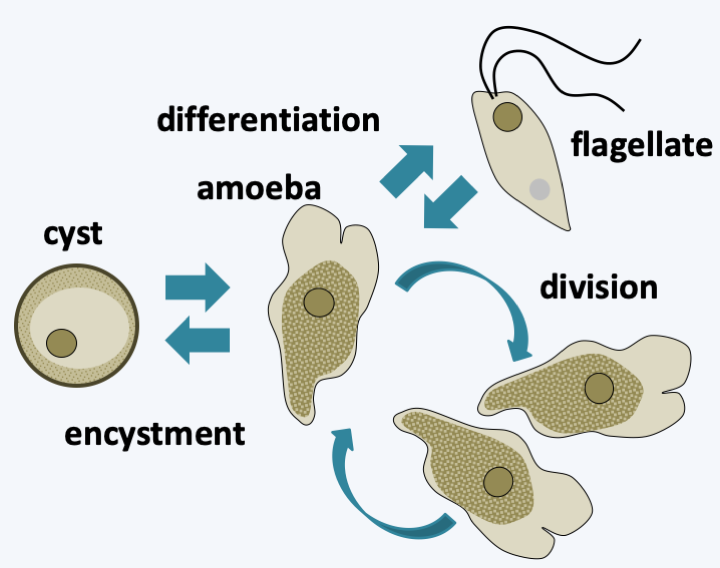
Figure 206.
Cycle of Naegleria gruberi. This species possesses the cyst and flagellate differentiation capabilities typical of the group. The differentiation of the flagellated amoeba is very rapid, lasting about an hour. It can be induced in the laboratory by diluting, for example, the amoeba culture medium. Only amoeba divides.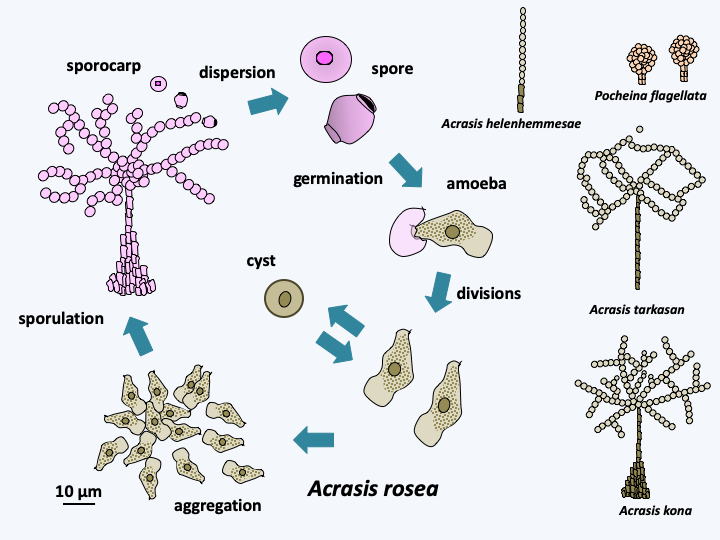
Figure 207.
Cycle of Acrasis rosea and sporocarps of other Acrasida species.Percolozoa
The classification of Percolozoa is constantly changing andFigure 198gives only a provisional phylogeny which is expected to change rapidly. Initially, the Heterolobosea phylum included non-aggregative amoebae in the class Schizopyrenida and aggregative ones in the class of Acrasida. Then other species, in particular Pseudociliatida of the genus Stephanopogon, have been shown to be related to Heterolobosea. Likewise, the Acrasida seem to derive from particular Schizopyrenida. Certain biologists then kept the name of Heterolobosea understood in the broad sense (sensu lato) to include all the species, while others opted for the new name of Percolozoa, keeping to the Heterolobosea in the strict sense (sensu stricto), but by changing its status to a class with two orders: Schizopyrenida and Acrasida. For these latter biologists, the genus Stephanopogon and the Percolomonas which are related to them are placed in another class, that of Percolatea. Unfortunately, molecular phylogenies are at odds with the ability of cells to aggregate, as well as the morphological data of the cyst and flagellar apparatus that were used to establish the traditional classification. Molecular phylogenies indicate that the species now included in the class Pharyngomonadea diverged first (Figure 198andFigure 205, Pharyngomonas kirbyi). These species have a biology typical of the group as they can alternate between an amoeba form and a swimming form with four flagella. The remaining species form an evolutionary radiation of at least six independent lineages whose branching order in the phylogenetic tree is unclear. Five of these lineages, all currently unnamed, overlap the old class of Heterolobosea, and the last one overlaps the old class of Percolatea. The Acrasida are included in one of the unnamed lineages. Apart from the synapomorphies of Percolatea which have lost the amoeboid form and live as flagellates or ciliates capable of encystment, there is no obvious shared character for the other lineages. All six lineages define the class of Tetramitia (Figure 198).
Euglenozoa
The second branch of the Discicristata, that of the Euglenozoa, groups together four classes (Figure 198). Based initially on molecular phylogeny, one trait turned out to be shared by all classes. Indeed, the expression of their genome seems very different from that in other eukaryotes. Genes are grouped into polycistronic transcription units like bacterial operons. However, these genes are not put together according to their function as in the case of prokaryotes. There is a trans-splicing mechanism that allows the long polycistronic pre-messenger RNA to be split into monocistronic mRNA (see Figure 15). Mature mRNA is then exported to the cytoplasm for translation. Analysis of the 5’ ends of the mRNA shows that they are identical for many genes. They are in fact added during the trans-splicing reaction between the pre-messenger RNA of the gene and a structured RNA common to many genes, SL RNA. This reaction is probably catalyzed by the splicesome. Despite their co-transcription, the mRNA of genes of the same unit are present in variable quantities, showing that the regulation of expression takes place largely at the level of the degradation of the mRNAs. Analysis of the promoters shows that they do not contain consensus sequences identical to typical eukaryotic promoters and that their functioning is therefore probably different. Analysis of the available genomes, which so far all belong to members of the Kinetoplastida class, shows an impact of this mechanism on the overall organization of genes: they are oriented in the same direction over very large chromosomal portions.
Diplonema and Symbiontida
Two classes, Diplonema and Symbiontida contain only a few species each (Figure 208). Symbiontida live in association with epibiotic bacteria in intertidal sediments poor in oxygen, to which they are sensitive. Due to their lifestyle, their mitochondria are modified and resemble hydrogenosomes. The lack of extensive sequence data on species in this class limits our knowledge of them. Their ribosomal DNA is easily amplified from samples of anoxic marine sediments, suggesting that they are common in these environments. Most Diplonemida are phagotrophs, but some species may be parasitic on diatoms or invertebrates at certain points in their life cycle. Although few species are described, they appear to be present and common in ocean waters, both near the surface and deeper in the ocean. While the exact position of Symbiontida within Euglenozoa is unclear, Diplonemida are closer to Kinetoplastida than to Euglenida. Indeed, the sequencing of the mitochondrial genomes of Euglenozoa shows that they are fragmented in Euglenida, Diplonemida and Kinetoplastida. However, the structures are different in the three groups. Diplonemida have a mitochondrial genome where genes are fragmented into pieces of about 250 base pairs and are embedded on small circular molecules, which strongly impacts the structure of mRNAs (see Box 5). In addition, some mRNAs are edited. In Kinetoplastida, the mitochondrial genome is also fragmented into circular molecules and the gene sequence has degenerated greatly, so the mRNAs must also be corrected by editing, often extensively (see Box 5). In contrast, in Euglenida, the mitochondrial genome appears to be fragmented on linear molecules and mRNAs do not require editing before being translated. In addition, Diplonemida and Kinetoplastida, but not Euglenida, exhibit compartmentalization of certain glycolysis enzymes in special peroxisomes, glycosomes, a unique feature among eukaryotes.
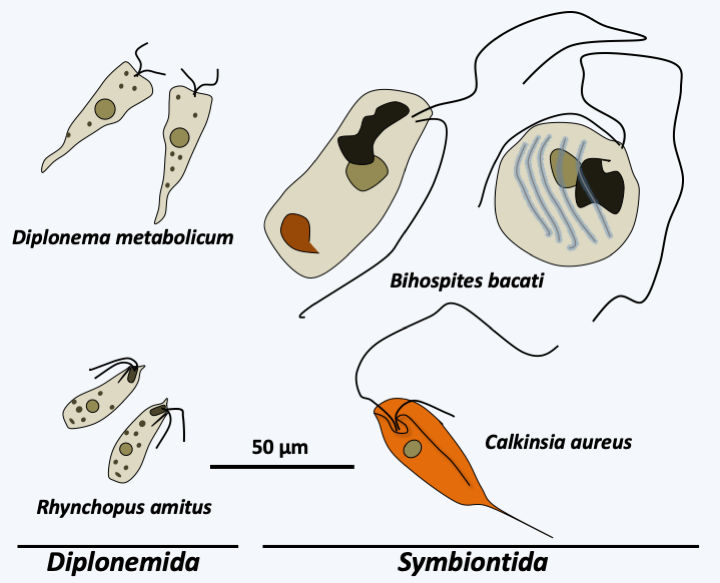
Figure 208.
Some Diplonemida and Symbiontida.Kinetoplastea
The class of Kinetoplastea, containing the unique order of Kinetoplastida, takes its name from the particular structure of the mitochondria found in the various members. This indeed contains an opaque inclusion, perfectly visible under a microscope, the kinetoplast. This is in fact the mitochondrial genome, whose fragmentation, amplification, and the typical pattern of expression of the class result in enough protein-complexed nucleic acids to be detectable under light microscopy. While the kinetoplast is present in all species of the group, its structure and function differ depending on the species. For example, different genes undergo editing to different degrees. Note that editing is regulated according to the life cycle stage. In human parasites which alternate between humans and an insect, mitochondrial respiration is active in the insect but inactive in humans; this is correlated with different editing levels for certain mRNAs.
There are about 400 species of Kinetoplastea (Figure 209). The typical vegetative form is monoflagellate or biflagellate, its morphology changes strongly during the life cycle. Some of the Kinetoplastea live as free phagotrophs, while others are parasites of other protists, plants or animals. Some have only one host while others have two. One species, Perkinsela sp., lives as an endosymbiont of amoebae of the genus Neoparamoeba, Amoebozoan parasites of salmonids. It has degenerated a lot and now resembles only an organelle affixed to the nucleus of the amoeba and dividing in a coordinated manner with it (Figure 210). Molecular phylogenies show that parasitic species derive from free species and that two-host parasitic species derive from one-host species. The most likely scenario is therefore early independent adaptations to single host parasitism in many Kinetoplastida lineages. The modalities of these adaptations can be varied. For example, Ichtyobodo are ectoparasites of fish, while Paratrypanosoma confusum is an intestinal parasite of Culex pipiens mosquitoes. Note that while this parasite seems common in mosquitoes, it has never been found in the blood of vertebrates, suggesting that it remains confined to the intestine and is not injected during the blood meal, which confirms its specific location in the mid and posterior intestines. If the first host feeds on another organism by biting it, it becomes a vector when the parasite adapts to infect a second host. In fact, vertebrate parasitic Kinetoplastea are transmitted by mosquitoes, sand flies which are bloodsucking flies, fleas or even leeches. Likewise, the Phytomonas which infect plants are transmitted by various phytophagous insects. The Leishmania, on the other hand, have a particular mode of transmission. They are present in the droppings of Reduviidae, which are blood-sucking bugs. It is not the bite that transmits the parasite, but the scratching of the pimples they cause in the presence of contaminated droppings. The phylogenetic position of Paratrypanosoma confusum confirms this mode of evolution from one- to two-host parasites because it diverges from the radiation base of Trypanosomatida, the largest family of Kinetoplastida containing only parasites, some of which are one-host and others two-host. It is in this family that the parasites that affect humans reside (Table 11). The best-known species of the group is Trypanosoma brucei (Figure 211) which causes sleeping sickness. This species is divided into three subspecies. The first, Trypanosoma brucei ssp. brucei, is not pathogenic to humans but infects animals. The second, Trypanosoma brucei ssp. gambiense, is responsible for the majority of cases and causes a rather chronic disease with a slow progression; this subspecies appears to be specific to humans. The third, Trypanosoma brucei ssp. rhodesiense, causes rapid and severe infection; this subspecies, obviously ill adapted to humans, usually infects animals. The life cycle alternates between the tsetse fly, in which the parasite reproduces sexually, and humans or animals (Figure 211). Currently, the epidemics of sleeping sickness, or African trypanosomiasis, are under control and the parasite “only” causes tens of thousands of deaths per year. Chagas disease, or American trypanosomiasis, causes the same number of deaths, but the parasite responsible, Trypanosoma cruzi, mainly chronically infects more than eight million people, in whom it causes heart or digestive problems. Leishmaniasis is caused by more than 20 different species and is mostly benign, but can often leave deforming scars. Visceral forms are rarer but fatal if left untreated. Currently, they cause more deaths than trypanosomiasis. These parasites unfortunately have animal reservoirs, which makes their eradication impossible.
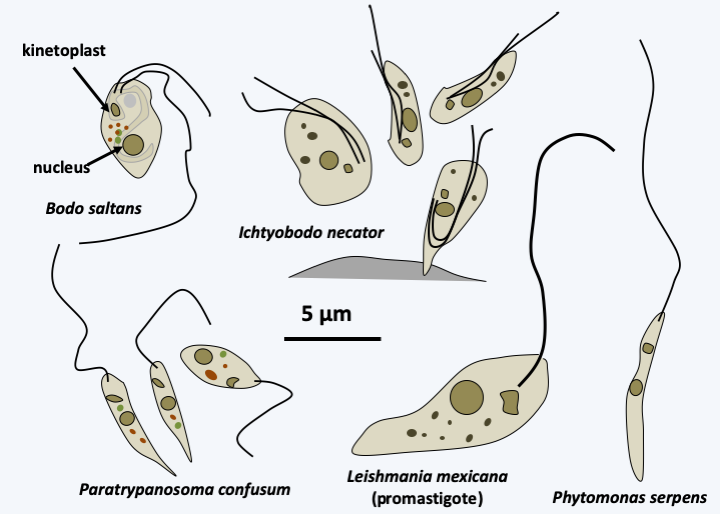
Figure 209.
Some Kinetoplastea.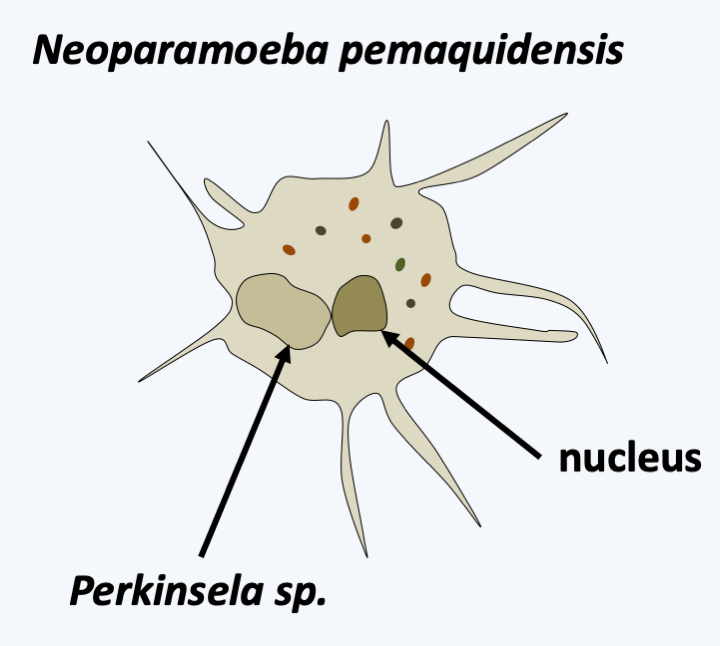
Figure 210.
Perkinsela sp., an endosymbiont of amoebae of the genus Neoparamoeba.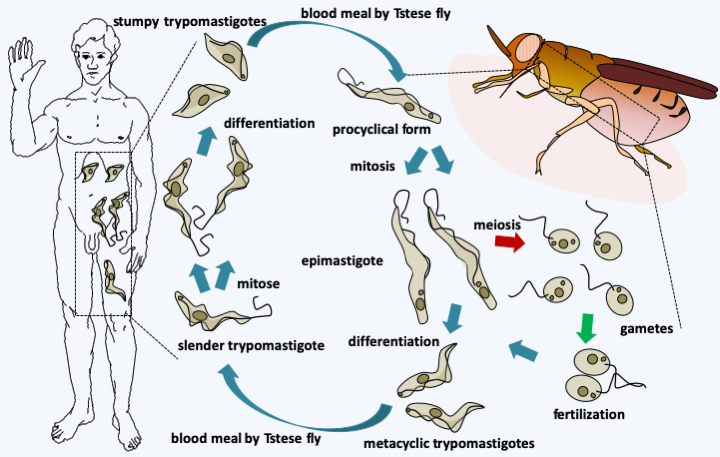
Figure 211.
Cycle of Trypanosoma brucei gambiense. The cycle begins with the tsetse fly eating a blood meal from an infected host. The stumpy trypomastigotes in the blood will differentiate into epimastigotes and migrate from the fly's intestine to its salivary glands. They will divide and eventually engage in meiosis. The haploid cells produced will immediately conjugate to give back diploid epimastigotes. Meiosis begins a dozen days after the parasites enter the fly and fertilization can be initiated by gametes from parasites taken from different blood meals, which contributes to the genesis of genetic diversity. Epimastigotes divide by mitosis and eventually differentiate into infecting metacyclic trypomastigotes which will be injected during another tsetse fly blood meal. Once in human blood, they differentiate into slender trypomastigotes which multiply by mitosis, until they differentiate into stumpy trypomastigotes.Euglenida
With more than 1,500 described species, the Euglenida class is numerically the most important among the Excavata. They are single-celled flagellates (Figure 212) with one or two flagella, rarely more. They most often inhabit freshwater, rich in organic matter in virtually all climates. Some species are marine. While most of them live free, a few, such as Colacium vesiculosum are epibionts or endobionts mainly of copepods at certain stages of their cycle. The flagella are inserted into a pear-shaped reservoir, the vestibular complex, invaginated inside the cell. The cell is strengthened by a flexible cortex called pellicle. This “skin” is made up of microtubules, specific proteins and invaginations of the endoplasmic reticulum, the whole organized in bands, the number of which varies according to the species. It allows cells to change shape in a process called metaboly (Figure 213), and strengthens the cell. Euglenida also have a cytostome, or cell mouth, supported by microtubules. The structure of the pellicle and the cytostome are different depending on the feeding patterns of different Euglenida. Indeed, some species are bacterivorous phagotrophs, others are “eukaryotivorous”, that is to say, consume mainly eukaryotic cells. Some eukaryotivores and parasites are able to feed by myzocytosis (see Figure 41). There are also osmotrophs and especially photosynthetic autotrophs which result from the secondary endosymbiosis of a Viridiplantae. The best-known species, Euglena gracilis (Figure 212), is one of these photosynthetic autotrophs; it is used in several laboratories to study the physiology of microalgae. Some species can adopt several of these nutrient modes depending on the environment. Molecular phylogenies offer a tree that allows us to better understand the evolution of different trophic modes and correlatively of morphology (Figure 214). At the base of the tree are the bacterivorous species which are inflexible because they have few bands, twelve at most. They have a well-defined cytostome, allowing them to phagocytize their prey. Eukaryotivores are generally larger and the more “complex” species are capable of metaboly and have a cytostome suitable for ingestion of larger prey. Their pellicle is made up of several dozen bands. A regression of the oral apparatus occurred in two lineages. The first resulted in osmotrophs living in waters very rich in organic matter. These species show regression of the pellicle and cytostome. The second is that of the photosynthetic Euglenida, the Euglenophyta. The earliest diverged species in the photosynthetic lineage, Rapaza viridis, has a mixotrophic diet. It has a plastid from secondary endosymbiosis with a Viridiplantae. But, despite its plastid, Rapaza viridis cannot survive more than 35 days in light and a week in the dark without phagocytizing prey using a still well-developed cytostome. The plastid of Rapaza viridis originated from “Tetraselmis sp. (PRA-361)”, a Viridiplantae of the class of Chlorophyta. Rapaza viridis is able to retain the plastids of Tetraselmis sp. (PRA-361) in its cytosol for a long time showing that it is undergoing kleptoplasty. The rest of the photoautotrophs have regression of the oral apparatus and pellicles. They have also acquired an ocellus which allows them to detect light (Figure 212). The plastids are surrounded by three membranes, which suggests that it was acquired by myzocytosis and kleptoplasty. Analysis of the chloroplast genomes of photosynthetic species shows that they contain particular so-called group III introns. Some of the osmotrophic species derive from photosynthetic species that have lost the functionality of the plastid. Euglenida therefore show a complex evolution of their characters in relation to the trophic modes adopted.
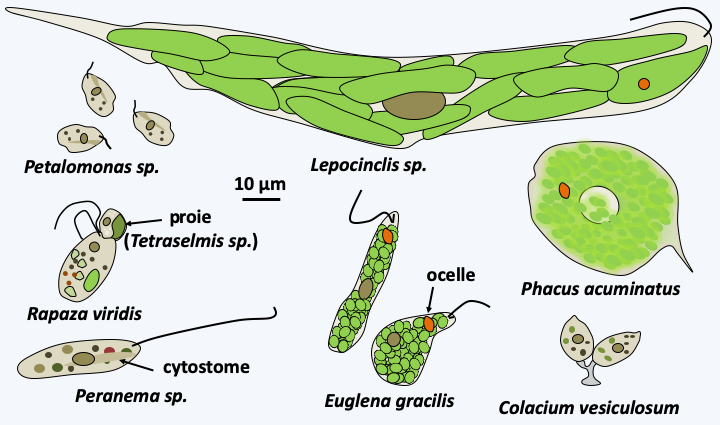
Figure 212.
Diversity of Euglenida.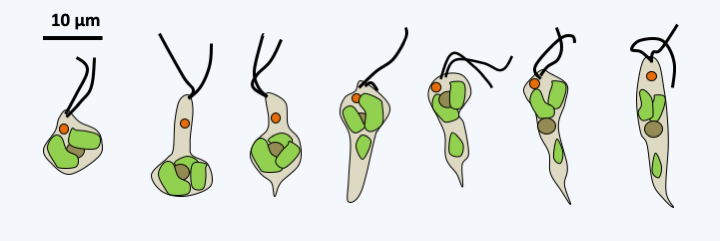
Figure 213.
Shape shifts in Rapaza viridis. The changes of shape are very fast. It takes a few seconds at most to switch from the left shape to the right shape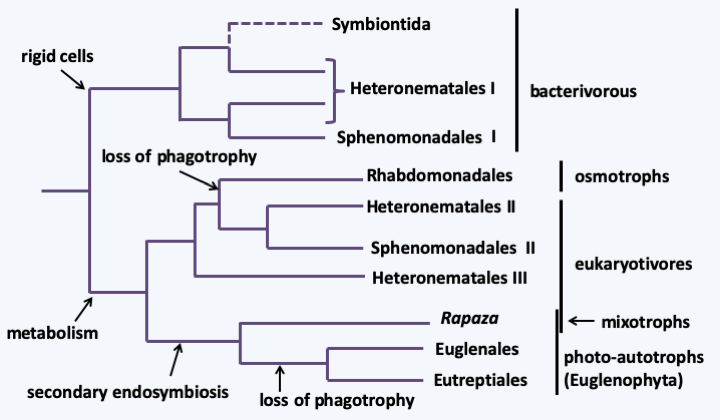
Figure 214.
Phylogeny of Euglenida.There are other characters unique to Euglenida. At the nucleus level, there appear to be two types of introns: introns of the 5’-GT..AG-3’ form usually found in other eukaryotes, and introns which diverge from this pattern. On the other hand, the latter are bordered by repeated sequences (which makes it difficult to determine their limits) and can form secondary structures which bring their 5’ end closer to their 3’ end. The splicing mechanism of these introns is not yet known. Another characteristic of Euglenida is the permanent condensation of their chromosomes. The chromosomes divide by closed mitosis, i.e. the nuclear membrane never disappears. Sexual reproduction has not been detected in Euglenida, and they are only known to divide by mitosis followed by longitudinal division of the cell. Beforehand, the pellicle bands, flagella and the oral apparatus are duplicated. Unlike Kinetoplastida, the absence of major pathogens in the class does little to encourage scientists to study these organisms. However, the complexity of their evolution shows that this is a clade with a very long evolutionary history, like the other Excavata lineages.
Back to chapter index